|
|
|
The Chemist Volume 91 | Number 1 |

|
|
|
Principles, Policies and Practices in establishing
a Post-Secondary Chemistry Department:
A retrospective evaluation
|
|
Abstract: A brief description of a successful time-tested chemistry department in an Australian university is presented. It is remarkable that the chemistry department in the La Trobe Institute of Molecular Sciences has generated international distinction and survived nearly a half-century as a cohesive, independent academic unit despite several university restructuring efforts [1]. Obviously, this department has shown resilience to many institutional forces of change and financial instabilities and remained committed to academic excellence. Hands-on laboratory based classes in practical and theoretical chemistry continue to generate graduates well-positioned to enter the twenty-first century professional work force in chemistry and its partner sciences.
Key Words: Chemistry Department, University, College, Post-Secondary, Policy, Practice, Evaluation.
|
|
|
Introduction
Chemistry has always been recognized as an essential discipline in university faculties, schools and colleges of science and is still widely regarded as the ‘central science’. The study of organic chemistry dates from the mid-eighteenth century. Inorganic chemistry had its origins in the early twentieth century and analytical and physical chemistry in the early to mid-twentieth century. Hence over the past 150 years, four major branches of chemistry have been recognized (Fig.1). In the late twentieth century, the number of chemistry branches exploded (Table 1) and chemistry enables many other ‘sciences’ (Fig. 2). Also, two major adjuncts of chemistry, ‘Chemical Education’ and ‘Chemistry in Society’, have emerged (Fig. 3) which not only promote and enrich the teaching of chemistry, but also emphasize the ‘relevance’ of chemistry in the developing world.
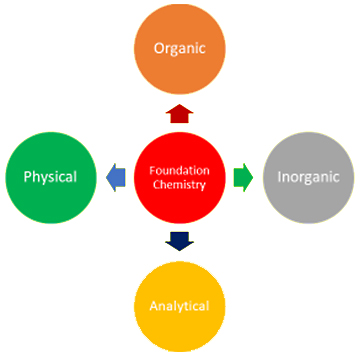
Fig 1. The four foundation braches of chemistry |
Most of university foundation chemistry departments around the world have prestigious records in terms of teaching and research and many of these remain as ‘stand-alone’ entities. It has always been recognized that academic science departments are expensive to establish and to maintain due to setting up teaching and research laboratories and, in particular, purchasing state of the art research instrumentation. Thus, in the late twentieth century, chemistry in many of the newer universities was amalgamated into compatible science departments such as biochemistry, physics, earth, life sciences and others and as a consequence became the minor component of these mega-departments. Although chemistry has always been recognized as the ‘central science’, financial realities forced these amalgamations coupled with a decline in students electing to study chemistry as their major. Also, many newer universities have not included chemistry as a foundation academic entity.
Table 1. The New Age Branches of Chemistry
|
Aquatic Chemistry |
Atmospheric Chemistry |
Biochemistry |
Chemical Kinetics |
Chemical Thermodynamics |
Computational Chemistry |
Coordination Chemistry |
Electrochemistry |
Environmental Chemistry |
Femtochemistry |
Food Chemistry |
Forensic Chemistry |
Geochemistry |
Green Chemistry |
Industrial Chemistry |
Macromolecular Chemistry |
Magnetochemistry |
Marine Chemistry |
Materials Chemistry |
Chemical Mathematics |
Medicinal Chemistry |
Molten Salt Chemistry |
Nanochemistry |
Natural Product Chemistry |
Nuclear Chemistry |
Organometallic Chemistry |
Pharmaceutical Chemistry |
Pollutant Chemistry |
Polymer
Chemistry |
Supramolecular Chemistry |
Surface Chemistry |
Theoretical Chemistry |
Thermochemistry |
|
|
|
The factors which lead to the survival of chemistry as a ‘stand-alone’ department are complex and inter-related. Paramount of these is that ‘teaching’ is ‘informed by research’ – meaning that all academic staff in the department should be research active and hence offer topical and challenging research projects which attract higher degree students. The department should be recognized as a world leader and hence a ‘center of excellence’ in at least one research area and staff must actively apply for external research funding. To support this level of research excellence, the department must have ‘state-of-the-art’ research instrumentation and support technical staff to support ‘cutting edge’ research in a variety of areas. Also, academic staff should be appointed not only on their teaching ability, but also on the topicality and relevance of their research interests and how these blend into a cohesive whole. Consistent with this is an assessment of their successful potential for the awarding of external funding. Also of importance is their potential to collaborate with researchers nationally and internationally.
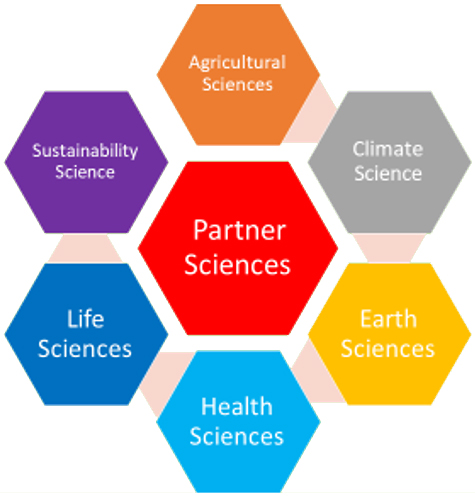
Fig 2. Chemistry partner sciences |
Although not always recognized as of critical importance, instructive teaching and learning strategies also characterize and enhance the ‘stand-alone’ longevity potential of a chemistry department. In recent times, chemistry departments have established ‘learning centers’ in which ‘state-of-the-art’ teaching and learning strategies are implemented with the direct involvement of students on an individual basis. Also, since chemistry is an experimental science, it is essential for students to undertake ‘hands-on’ laboratory exercises during all 3 years of their course. There are a plethora of ways in which these experiments can be intermeshed with the teaching program and in the final year, it is usual to design experiments which introduce students to the principles and practices of research. In essence, the teaching and learning and research metrics contribute equally to the long-term success of a chemistry department.
As an example of the best principles, practicalities and policies being applied to establish a post-secondary chemistry department, La Trobe University in the State of Victoria, Australia was established in 1967 and was the third University to be established in Melbourne after Melbourne and Monash Universities and La Trobe Chemistry was a foundation department in the School of Physical Sciences. As part of the golden jubilee celebrations of La Trobe University in 2017, the 50-year history of La Trobe Chemistry has been published as an e-book [1] and its ‘stand-alone’ status throughout this period is testament to the effectiveness of the principles and policies on which it was founded.
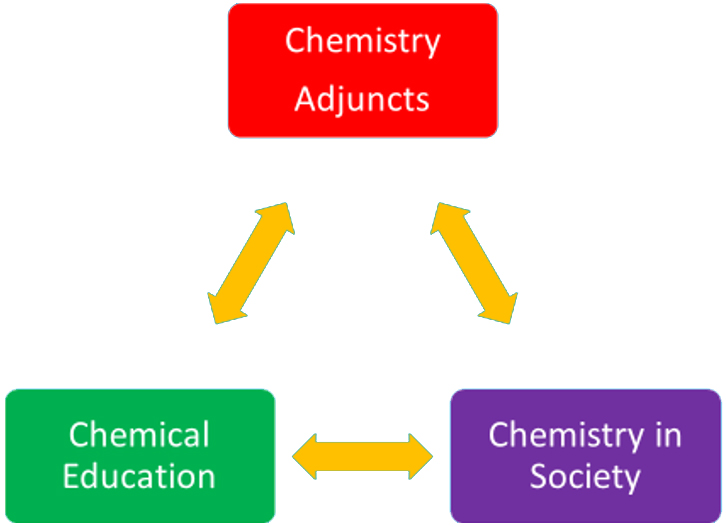
Fig 3. Chemistry adjuncts |
Initially, La Trobe Chemistry consisted of three ‘divisions’, Organic, Physical and Inorganic. The latter was expanded to ‘Inorganic and Analytical’ in the 1980’s. A Professor headed each of these divisions and foundation academic staffs were appointed on the basis of having a higher degree (most had a PhD) and being research active. A further consideration was that appointees would collectively provide a range of research fields in each of these three divisions, which as far as possible would not duplicate those at Melbourne and Monash Departments of Chemistry. Thus, Physical Chemistry concentrated on mass spectrometry, X-Ray crystallography, gas phase kinetics and later, photoelectron spectroscopy. The co-establishment of well-equipped mechanical, glass and electronic workshops enabled some of this equipment to be built ‘in-house’ since at this time, commercial counterparts were not available. Melbourne and Monash Chemistry Departments were strong in ‘natural product synthesis’ so La Trobe Organic Chemistry concentrated on ‘physical organic chemistry’ as its main research theme, with emphasis on the mechanisms of organic reactions. However, there was also research on the synthesis and characterization of heterocyclic compounds and drug design and development. Infrared and UV-Visible spectroscopy and H-1 NMR instrumentation was available in house to support this research. Foundation staff in the Inorganic Division had research interests in both inorganic and analytical areas and included coordination chemistry, solvent extraction, ion-selective electrodes, electrochemistry, gas liquid chromatography, molten salt chemistry and environmental chemistry. Subsequent academic appointments in the 1980’s and 1990’s added considerably to the range of research fields in all three divisions and included multi-nuclear NMR leading to the establishment of an ‘NMR center of excellence’, development of new anti-cancer drugs using molecular graphics, organic chemical kinetics and the effect of micelles on catalytic processes, development of flow analytical methods, especially Flow Injection Analysis, use of multi-nuclear NMR, especially Mo-95 to study ‘bi-nuclear reaction centers’, and the role of molybdenum in biological systems. By the end of the 1980’s, La Trobe Chemistry had become a ‘stand-alone’ department and had several research centers of excellence. In addition to the NMR center, these included mass spectrometry, X-Ray crystallography, bioinorganic chemistry and Center for Scientific Instrumentation. By the end of the twentieth century, most of the first generation of academic staff had left through retirements and hence a next generation of academic staff was appointed which brought ‘frontier chemistry’ research projects into La Trobe Chemistry. These included medicinal chemistry, supramolecular chemistry, computer design of molecules in conjunction with prediction of their properties, molecular sensing and development of new analytical techniques capable of ‘ultra-low’ detection limits, nano-structures, polymer inclusion membranes, study of inter-stellar nano-particles using the infra-red beam line of a synchrotron, investigation of nanostructures for applications in electronic devices, gas sensors and batteries and computational chemistry with emphasis on predicting ‘new chemistry’, modelling reaction mechanisms, and studying metal-containing systems which phosphoresce and luminesce.
Over the 50-year period of its existence, La Trobe Chemistry has a record to be reckoned with in terms of its research output, as measured by the numbers of Honors and Higher Degree graduates, the continuing establishment of internationally recognized research centers of excellence, the successful awarding of external competitive research funding and the number of peer-reviewed publications produced. The founding principles and strategies for setting up the Department as a research active entity have been endorsed and continue to be implemented.
La Trobe Chemistry also has a distinguished teaching and learning record to be reckoned with based on the ‘teaching informed by research’ founding ethos of the University established 50 years ago. ‘Year 1 Chemistry’ has always been subdivided into two streams. In the early decades, these were known as ‘Chemistry 1A’ and ‘Chemistry 1B’. The former was for students who had passed chemistry at the Higher School Certificate (HSC) level and who were likely to study chemistry as their major, whereas the former was for students who were enrolled in other sciences such as biology and agriculture. In later decades, ‘Year 1 Chemistry’ was restructured into two streams (‘Basic Chemistry’ and ‘General Chemistry’) in Semester 1 and ‘Applied Chemistry’ in Semester 2. The latter course was available for students who had passed ‘Chemistry General’ in Semester 1. From inception, it was decided that three laboratory sessions per week in Semester 1 in each of the three main branches of chemistry were assessed components of ‘Year 1 Chemistry’ in order to convince students that ‘chemistry’ was fundamentally an ‘experimental science’ and ‘theories’ had to be confirmed by ‘experimentation’. In recent decades, a ‘Bridging Chemistry course’ was made available to assist students with limited background knowledge of chemistry to prepare for Chemistry 1 courses and a ‘Learning Resource Center’ was established ‘in-house’ for enriching and enhancing the student learning experience. From the early 1990’s, the Chemistry 1 course was made available online in ‘modular’ format – thus keeping abreast of ever-advancing teaching and learning strategies and conforming to ‘chemical education’ philosophies and practicalities of teaching and learning chemistry.
‘Chemistry 2’ was also initially constructed in two streams which built of the basic concepts of chemistry introduced in ‘Chemistry 1’ and applied these to expand the chemistry knowledge of students. For example, in organic chemistry, synthesis methodologies and organic reaction kinetics and mechanisms were emphasized in conjunction with the principles of the emerging diagnostic NMR technique. In inorganic chemistry, coordination and ‘p-block element’ chemistry were introduced together with the principles of the major characterization techniques of infrared and UV-Visible spectroscopy, conductance and magnetometry. For physical chemistry, surface chemistry and the emerging instrumental techniques such as mass spectrometry, molecular spectroscopy and gas phase chemistry were introduced. As for ‘Chemistry 1’, weekly practical sessions in the three main braches of chemistry were compulsory, which for organic and inorganic chemistry were focused on ‘synthesis’ in conjunction with ‘characterization’ using the major spectroscopic techniques. Thus, much emphasis was placed on students having ‘hands-on’ experience with common and ‘emerging’ laboratory instrumentation such as ‘NMR’.
‘Chemistry 3’ continued with the two stream format with the B stream having an applied/industrial chemistry emphasis, while the A stream tended to focus on ‘emerging chemistry’ concepts and introduce the principles and significance of research. ‘Chemistry 3’ also included weekly laboratory sessions in each branch of chemistry which involved multi-faceted advanced experiments in conjunction with access to ‘hands-on’ experience with advanced instrumentation such as NMR, HPLC, Thermal Analysis, MS and ESR. The ‘Chemistry 3’ laboratory program essentially consisted of a series of ‘mini-projects’ which were undertaken by student pairs. The ‘Chemistry 3B’ course also included guest lecturers, largely from the chemical industry who gave an insight into their specialist areas and also an overview of career opportunities available to chemistry graduates. This gave students an insight into ‘chemistry in the real world’. Recent ‘Chemistry 3A’ courses of special interest were ‘Molecular Design’ and ‘Fuels, Energy and Environmental Sustainability’. The former was a completely computerized course showing how ‘molecules’ can be ‘designed’ to have ‘pre-specified’ properties and the latter addressed the paradox that the continued combustion of fossil fuels to produce energy is unsustainable if the natural environment is to survive the consequences of global warming.
The ‘Chemistry Honors’ course (4th year of the conventional BSc degree) continues to be based on a series of lectures on ‘specialist and leading edge’ chemistry topics plus a minor (research) thesis, the former being assessed by conventional examinations and the latter by the ‘supervisor’ and by oral defense. Recent honors lecture topics have included ‘medicinal chemistry’, ‘supramolecular chemistry’, ‘molecular design’, ‘molecular sensing’ and ‘computational chemistry’ with complementary honors research projects being available in these areas of specialization. Also, in recent decades, honors projects have been designed and co-supervised by personnel from external scientific organizations such as the ‘State Forensic Science Laboratory’, the ‘Environment Protection Authority’ and the CSIRO (various divisions). These collaborations have often led to employment for La Trobe Chemistry honors and higher degree graduates.
‘Quality Assurance’ has consistently featured prominently in establishing benchmarks and for setting standards for teaching and learning throughout the University and student assessment of teaching has become a routine metric of quality in this domain. La Trobe Chemistry has enthusiastically embraced and implemented these initiatives and the Department as a whole has consistently been acclaimed and rewarded both in the promotion process and more recently by competitive University and State Government citations and awards for its commitment and progressive achievements in the teaching and learning domain. La Trobe Chemistry has a distinguished and sustained record of performance excellence in its cumulative teaching and learning portfolio. In this context, it should be emphasized that steps to maintain quality control have implications for accreditation. In the United States college level chemistry, the American Chemical Society (ACS) accredits programs. The lessons learned from establishing benchmarks and setting standards at the La Trobe Chemistry Department provide insights into the inner workings of a successful time-tested chemistry department which are invaluable to accrediting agencies everywhere.
The viability of an academic chemistry department is dependent on a sustainable annual enrollment of undergraduate and higher degree students and hence it is vital that it continuously strive to attract students to undertake its courses. Furthermore, potential students assess tertiary courses not only on their academic credibility but on their cumulative ability to provide a viable platform for future employment. La Trobe Chemistry has throughout its existence made enthusiastic contributions to annual University Open Days by not only offering ‘show and tell’ sessions to visitors but also by entertaining them with the ever popular ‘Chemistry Magic Show’. Also, interactions with local secondary colleges have been established by inviting Years 10, 11 and 12 ‘science students’ from local schools to be involved in a ‘High-School Enrichment Program’ in the University chemistry laboratories – using equipment and instrumentation not usually available to them at school level. In recent years, La Trobe Chemistry has introduced ‘NMR learning skills’ into this program.
In terms of relating its courses to ‘career opportunities’, La Trobe Chemistry has consistently created and sustained interactions with the chemical industry and Government (scientific) institutions such as the Environment Protection Authority (EPA), CSIRO, National Forensic Science Laboratory and a variety of chemical industries, including the pharmaceutical and the emerging renewable energy industries.
In summary, perhaps the most remarkable achievement of La Trobe Chemistry is that it has survived as a cohesive, independent academic unit for nearly a half-century, when most academic chemistry departments in Australia have either amalgamated with other science departments or closed down. La Trobe Chemistry has continuously demonstrated its sustainability since its foundation despite many university restructuring efforts and continuous financial and infrastructure constraints. Its achievements over the last fifty years, as measured by its quality staff, research publication output, number and financial value of competitive external and internal research grants awarded and the number of higher degree graduates produced, testify to the overall resilience of the department to external and internal forces of change and a determination to survive and develop via passionate commitment to academic excellence. It has re-engineered and restructured its courses strategically and successfully to incorporate the emerging trends in teaching chemistry to meet societal needs [2]. Its history thus far foreshadows it is well placed in terms of moving towards a sustainable and rewarding future and that it is able to retain its leadership status among academic departments of the University in conjunction with continuing recognition of its academic excellence both nationally and internationally.
Acknowledgments
This paper is an abridged version and a retrospective evaluation of "Brownlee R, Hill J," La Trobe Chemistry: The first 50 years’ which was a contribution to the La Trobe University 50th Anniversary archive project 2017.
References
- Brownlee R, Hill J in La Trobe Chemistry: The First 50 Years, La Trobe University, Australia, 2017. e-Book: https://doi.org/10.4225/22/59fba954a0f4c
- Kumar DD. Trends in Postsecondary Science in the United States. Ann. Am. Acad. Polit. Soc. Sci., 2003, 585(1), 124-133.
|