|
|
|
The Chemist Volume 93 | Number 1 |

|
|
Chemical Characterization, Preparation of Biosurfactant and Biochemical Evaluation of Seed Oil of Luffa aegyptiaca
|
|
Abstract: This study aimed to provide insight to minimize domestic and industrial competition for vegetable oil while limiting the discharge of undegradable synthetic surfactants (pollutants) to the environment by exploring the potential of underutilized sponge seeds. Extraction and characterization of the seed oil were performed using standard procedures by American Oil Chemist Society (AOCS). Fourier transform infrared spectro-scopy (FT-IR) was used to characterize the prepared biosurfactant. Four antioxidant assays, namely 2,2′-Azinobis-(3-Ethylbenzthiazolin-6-Sulfonic Acid (ABTS), 1,1-Diphenyl-2-picrylhydrazyl (DPPH), Ferric Reduction Potential (FRAP) and Nitric oxide (NO), were used to determine the antioxidant potential of the seed oil and biosurfactant using gallic acid and sodium lauryl sulfonate (SLS) as standards. The biosurfactant shows appreciable efficiency in a salt tolerance level of 190 ppm above the range of 120 – 180 ppm recommended for hard water. The standard synthetic surfactant SLS shows a salt tolerance of 220 ppm. The clear absence of a hydroxyl group (O-H) in the seed oil and carbonyl group (C=O) in diethanolamine (surfactant), which were more prominent in the biosurfactant at 1618 cm-1, indicate the formation of a biosurfactant. Antioxidant analysis shows that oil has moderate antioxidant potential when compared to gallic acid, while the biosurfactant shows better antioxidant potential against ABTS radicals, DPPH radicals, and FRAP than SLS. SLS indicated improved nitric oxide (NO) antioxidant compared with the biosurfactant. This suggests that the prepared degradable biosurfactant has the potential to replace its synthetic counterparts, thereby mitigating environmental pollution. Biosurfactants with antioxidant properties can serve as additives to protect against oxidative degradation in allied products.
Key Words: biosurfactants, antioxidants, physicochemical, GC-MS characterization, organic chemistry/natural products
|
|
|
Introduction
Biosurfactants are amphiphilic surface-active agents with enhanced stability, moderate toxicity and highly degradable properties compared to their synthetic alternatives [1]. In recent times, there has been increased interest in the production of biosurfactants from renewable sources as a result of their advantages over synthetic surfactants with respect to renewability, availability and environmental friendliness. The lower application of biosurfactants is primarily attributable to the cost of production, among other things. However, the negative impact of continuous accumulation of chemical surfactants on the environment makes the renewed search for better eco-friendly surfactants an inevitable option [2-4].
Masakoralaet al. [5] reported that the absorption of synthetic surfactants significantly reduces the ability of plants to convert photochemical energy from the sun. Similarly, the growth of lettuce was reportedly affected as a result of synthetic surfactant, as stated by Johnsonet al. [6]. Potential applications of biosurfactants include enhanced oil recovery [7], bioremediation processes owing to their potential to emulsify contaminants [8], and stabilization of food as a result of their anti-oxidant potential [9].
Growing interest in naturally preserved foods has further necessitated research into surfactants of fat and oil origin that are capable of abolishing the use of synthetic surfactants [10]. These other reasons are why this research on the properties and antioxidant potential of biosurfactants produced from seed oil of sponge seeds was carried out.
Sponge seed “Luffa aegyptiaca” is a subtropical vegetable and widely cultivated plant in tropical and subtropical countries. In many countries, the plant grows as a weed, particularly in Nigeria, where it is often thrown away as waste as the fibre part is much desired as a natural sponge. Stephens [11] reported that the plant contains both medicinal and nutritional agents and has been used for cleaning, filtering, and bathing. Balakrishnan and Huria [12] reported the pharmacological activities of L. cylindrica, another common species of the plant, which include antiplasmodial and antibacterial activities. In another study, the antihyperglycemic and antihyperlipidemic activities of ethanolic and aqueous extracts of the fruit of L. aegyptiaca were attributed to the presence of various phytochemicals, such as alkaloids, steroids, triterpinoids, flavonoids, and glycosides [13].
This work focused on the preparation, physicochemical properties, characterization, and antioxidant properties of diethanolamide from underutilized sponge seeds.
Materials and Methods
A fully matured sample of sponge seed was collected from Ogbondoroko, Afon area of Kwara State, Nigeria. The plant material was identified at the Herbarium of Plant Biology, University of Ilorin, Ilorin, Nigeria, where a voucher specimen was deposited. The seeds were deshelled, pulverized, dried, and kept in a cool dark place until needed for analysis.
Oil extraction was carried out using n-hexane (1000 mL) according to the method of Zubairet al. [14]. A 500 g sample material of pulverized dried seeds was extracted using a Soxhlet extractor at 55°C for 7 hours. The oil was obtained using a rotary evaporator at 40°C. Physicochemical properties such as acid value, iodine value, saponification value, peroxide value, and free fatty acids were determined using AOCS methods as described by Zubair et al. [14].
Fatty acid methyl ester was prepared according to the method of Zubair et al. [14]. 50 g oil was refluxed for one hour with 0.2 M methanolic HCl. At the end of the reflux, the FAMEs were obtained using n-hexane and concentrated using a rotary evaporator.
This was carried out following the method of Adewuyiet al. [15]. The reaction was carried out in a round bottom Pyrex glass. The reaction flask was equipped with a mechanical stirrer, thermometer and condenser. Esterified sponge seed oil and diethanolamine were reacted at a molar ratio of 1:6 in the presence of sodium methoxide as a catalyst (2% by weight of diethanolamine and oil). The reaction was carried out at a temperature of 115°C, while the formation of diethanolamine was monitored with TLC. At the end of the reaction, the mixture was allowed to cool and later dissolved in diethyl ether to recover the biosurfactant, and separation was performed using a separating funnel. The ether phase was washed with water and passed over sodium sulfate. The resulting ether fraction was later concentrated using a rotary evaporator. The scheme of the reaction is shown in Scheme 1.
 |
The esterified oil from the seeds was analysed using a gas chromatograph (6890N, Agilent Technologies Network) coupled to an Agilent technology inert mass selective detector (MSD) (5975B, Agilent Technologies Inc., Palo Alto, CA). Constituents were identified primarily based on the comparison of retention time with those of the authentic standards and further confirmed by comparing mass fragmentation patterns with those of the NIST library [14].
The infrared spectra were recorded on a Shimadzu 8400s according to the methods of Jainet al. [16] using a KBr pellet. The seed oil, diethanolamine and biosurfactant were each subjected to infrared spectroscopic analyses separately to identify associated functional groups.
Four antioxidant assays were used to determine the antioxidant potential of the seed oil, biosurfactants and the controls (gallic acid and sodium lauryl sulfonate).
This test was measured as described by Nishaaet al. [17] with little modification. The stock solution of the oil was prepared in dichloromethane to achieve a concentration of 100 µg/ml. Dilutions were made to obtain concentrations of 50, 25, 12.5, 6.25, 3.125, 1.562, 0.781, 0.390, 0.195 and 0.098 µg/ml. Two milliliters of each of the diluted solutions was added to 2 ml of a DPPH solution (10 µg/mL). It was further incubated for 30 min of reaction in a dark compound. Absorbance of the solutions was taking at 517 nm. The DPPH radical scavenging activity was calculated using the following formula (equation 1):
where A0 is the absorbance of the control and A1 is the absorbance of the sample.
The 2,2′-Azinobis-(3-Ethylbenzthiazolin-6-Sulfonic Acid (ABTS) antioxidant potential of seed oil, biosurfactant and sodium lauryl sulfonate (SLS) surfactant used as a control was established by reacting 2 mL of various concentrations 50, 25, 12.5, 6.25, 3.125, 1.562, 0.781, and 0.391 µg/ml of the samples with 2 mL ABTS cation solution following a standard assay procedure by mixing freshly prepared ABTS+ standard solution (7 mM). with potassium persulfate (2.45 mM). The resultant mixture was kept in the dark for 12 hours at room temperature, after which it was further diluted with methanol to achieve an optical density of 0.7 ± 0.01 when measured on a UV spectrophotometer at 734 nm. The ABTS solution will be allowed to react for 60 sec with various concentrations of the oil, biosurfactant and control surfactant, and the optical density will be read at the same wavenumber. Garlic acid was used as a standard control, and the experiment was carried out in triplicate [18].
The inhibition percentage of ABTS radicals was calculated using the following formula (equation 2):
where A0 is the absorbance of the control and A1 is the absorbance of the sample.
The Nitric oxide (NO) scavenging activity was determined according to the methods of Dashet al. [19]. Samples of different concentrations of 50, 25, 12.5, 6.25, 3.125, 1.562, 0.781, and 0.391 µg/ml were pre-pared. Griess reagent was prepared by mixing the same amounts of 1% sulphanilamide in 2.5% phosphoric acid and 0.1% naphthyl ethylene diamine dihydrochloride in 2.5% phosphoric acid immediately before use. A volume of 0.5 mL of 10 mM sodium nitroprusside in phosphate buffered saline was mixed with 1 mL of the different concentrations of the samples and incubated at 25°C for 180 mins. The samples were then mixed with an equal volume of freshly prepared Griess reagent. Gallic and SLS were used as control samples. The absorbance was measured at 546 nm using a UV-visible spectrophotometer. Inhibition was calculated (equation 3) as:
where A0 is the absorbance of the control and A1 is the absorbance of the sample.
Gangwaret al. [20] reported method was adopted for the FRAP assay. The reagent was activated by mixing 300 mM sodium acetate buffer (pH 3.6), 10.0 mM (tripyridyl triazine) solution and 20.0 mM FeCl3.6H2O solution in a ratio of 10:1:1 in volume. Two milliliters of each sample at different concentrations (50, 25, 12.5, 6.25, 3.125, 1.562, 0.781, and 0.391 µg/ml) was then added to 2 ml of FRAP reagent, and the reaction mixtures were incubated at 37°C for 30 min. Absorbance was measured at 593 nm spectrophotometrically. Fresh working solutions of FeSO4 were used for the calibration curve. The antioxidant potential was calculated from the linear calibration curve and expressed as mmol equivalents of FeSO4 per gram of sample.
Results and Discussion
Biosurfactants are amphiphilic substances with a wide range of applications. Their renewable nature made them perfectly fit to replace synthetic counterparts that are often attributed to as source of environmental pollution [6]. There is a wide range of underutilized seeds in tropical countries, such as Nigeria, that produce year in, year out without being put to appropriate use. These seeds are wasted beside the fact that the seeds themselves constitute environmental nuisance. Therefore, of the exploration of these underutilized tropical seeds as sources of raw materials in preparing biosurfactants can help in getting rid or minimizing environmental pollution.
The physical and chemical properties of the oil are presented in Table 1. Seed oil shows viability for use as an industrial source of raw material owing to its high oil yield of 25.96 ± 0.47%. The high acid value of 84.15±0.58 mg KOH/g indicates that the seed oil is not edible, as it is more than the recommended value for edible oil (4 mg KOH/g) by the WHO/FAO [14] and should be explored as raw materials for vegetable oil-dependent companies. Sponge seed oil shows a free fatty acid content of 1.09±0.01 mg KOH/g, a clear indicator that the oil will have a long shelf-life and stability to autooxidation [21]. The low free fatty acid content may be due to the low peroxide value of 3.95±0.14 meq kg-1, which indicates the presence of natural phytochemicals present in the oil preventing the oxidation and hydrolysis of the oil and hence the low free fatty acids. The high iodine value of 165.07±0.85 I2100 g-1 of oil exhibited by the seed oil indicates a high degree of unsaturation by the oil. The oil was liquid at ambient temperature, and the high degree of unsaturation was further confirmed by gas chromatography-mass spectrometry (GC-MS), as indicated in Table 2, with a total degree of unsaturation calculated to be 70.23%.
|
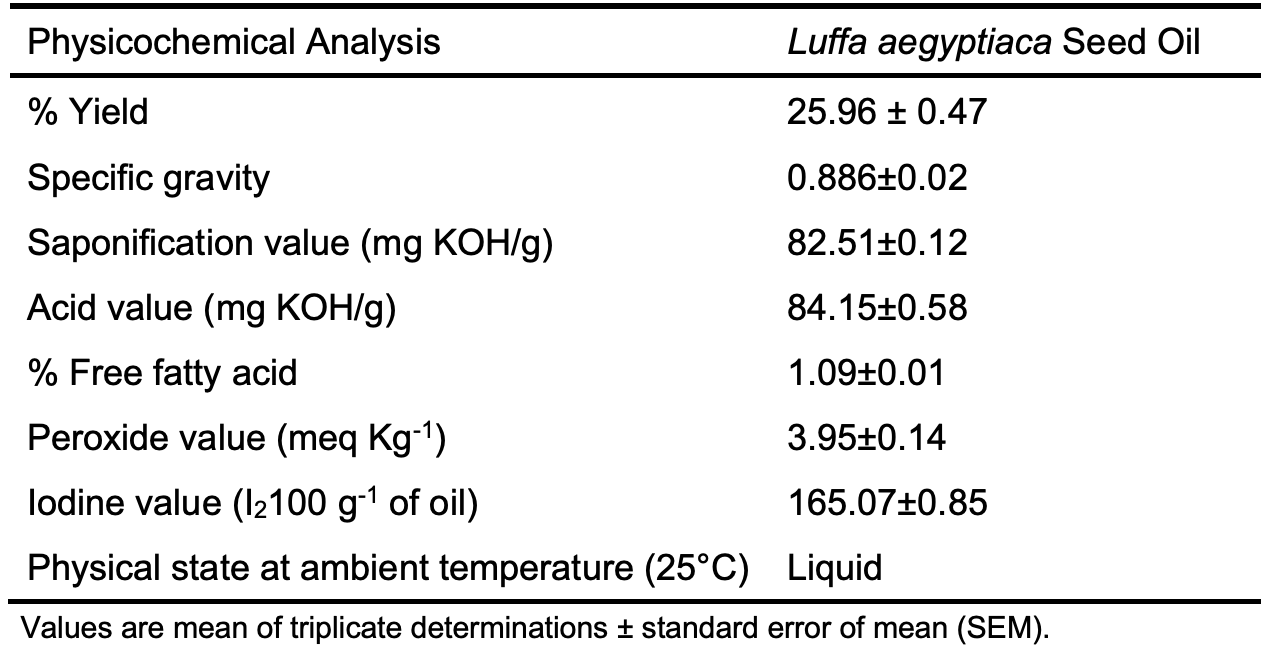 |
|
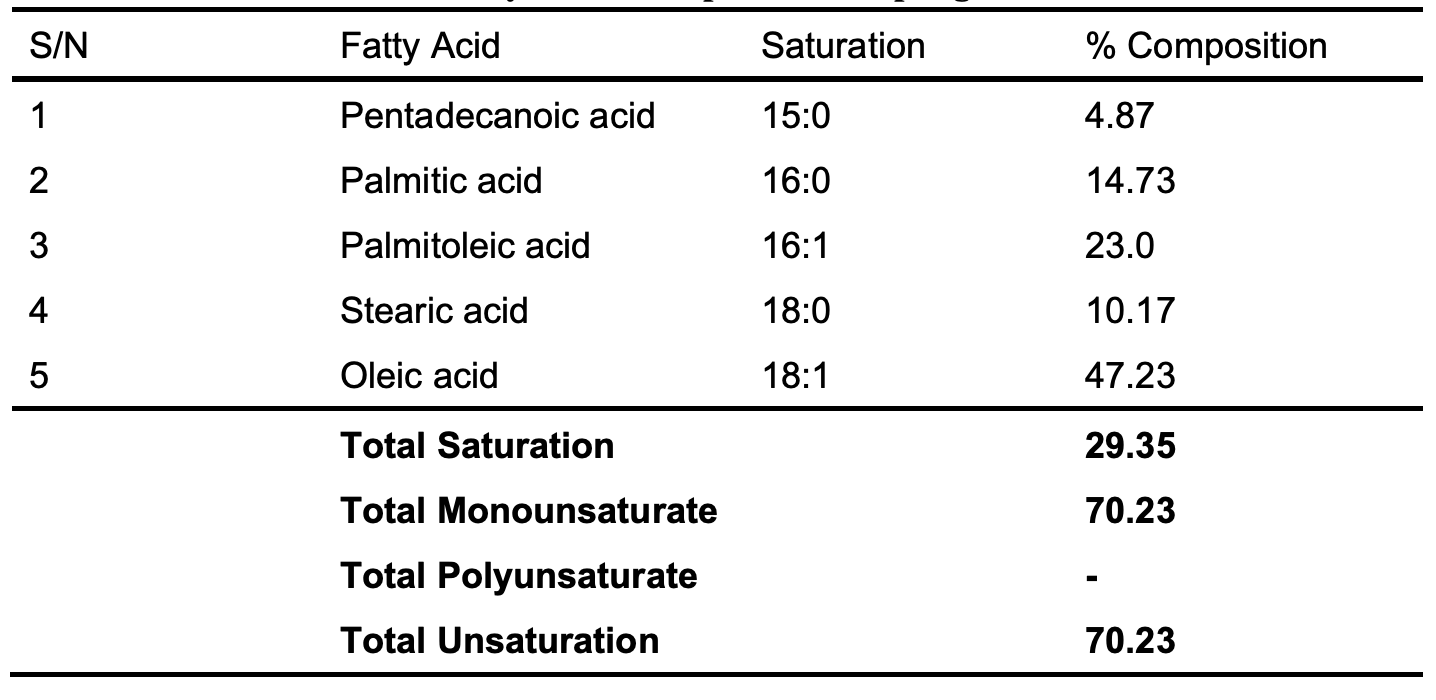 |
The fatty acid composition of the oil obtained from GC-MS is shown in Table 2. The most abundant fatty acids are oleic acid, a monounsaturated fatty acid that accounts for 47.23% of the total oil, followed by palmitoleic acid, another monounsaturated fatty acid that accounts for 23.0%, and stearic and palmitic acid, which both accounts for 10.17 and 14.73 degrees, respectively. The oil is largely made of unsaturated fatty acids.
The FT-IR spectra of sponge seed oil, biosurfactant and diethanolamine (super-imposed) are shown in Figure 1 below.
Table 3 shows deductions from the FT-IR spectra.
The results obtained and the emulsion stability test in Table 4 confirmed the preparation of the biosurfactant. The clear absence of a hydroxyl group (O-H) in the seed oil, whose presence was confirmed in the diethanolamine and the biosurfactant at 3394 cm-1 and 3404 cm-1, respectively, implied a possible difference in functional groups between the seed oil and the two other substances. Biosurfactant was also confirmed to have been produced by the absence of a carbonyl group (C=O) in diethanolamine, which was more prominent in the biosurfactant at 1618.33 cm-1. The prepared biosurfactant was further confirmed by comparing its emulsion stability to sodium lauryl sulfonate (SLS), as shown in Table 4. The biosurfactant shows a lower emulsion stability of 87.50 ± 2.53 seconds to 394 ± 1.41 seconds for SLS; however, the foaming stability shown by the biosurfactant for SLS was appreciable at 202.5 ± 3.44 cm to 250 ± 11.31 cm. The biosurfactant shows a greater ability to be effective in hard water, as it shows a salt tolerance level of 220 ppm well above the range of 120 – 180 ppm for hard water, as stipulated by the US Water Corporation. SLS shows a salt tolerance of 190 ppm.
One of the key functions of biosurfactants is corrosion inhibition, which is helped by their ability to resist oxidation, thereby offering protection to surface covers. The potential of the oil and biosurfactant produce to be used as an additive in corrosion inhibition was determined using four different in vitro antioxidant assays, and the results are reported below.
Table 5a shows the results of the ABTS Radical Antioxidant Potential Luffa aegyptiaca seed oil. Gallic acid was used as possible control. Figure 2a shows the graphical plots of the concentration in µg/mL and % inhibition from which the IC50 values were calculated.
The results showed a concentration-dependent antioxidant potential, and the highest inhibition potential was recorded at 100 µg/mL for both the oil and gallic acid, where the oil showed a maximum inhibition potential of 21.91 ± 0.022 compared to 94.33 ± 0.002 for gallic acid against ABTS radicals. The seed oil shows a far higher IC50 value of 46.06 µg/mL compared to 2.98 µg/mL, indicating that gallic acid has superior ABTS radical scavenging antioxidant potential than Luffa aegyptiaca.
Similarly, Table 5b shows the result of the DPPH Radical Antioxidant Assay L. aegyptiaca seed oil, with a dose-dependent inhibition range of 15.55 ± 0.002 - 7.63 ± 0.004 for L. aegyptiaca seed oil and 89.50 ± 0.006 - 29.70 ± 0.018% for gallic acid.
The IC50 value obtained for the DPPH assay from Figure 2b further indicates that gallic acid has better antioxidant potential than seed oil, 28.60 and 1.62 for L. aegyptiaca seed oil and gallic acid, respectively. Persea americana seed oil also shows limited DPPH antioxidant capability compared to gallic acid, as reported by Adaramolaet al. [22].
Table 5c shows the results of the ferric reduction antioxidant potential (FRAP) of Luffa aegyptiaca seed oil across a con-centration range of 100 to 1.563 µg/ml. The seed oil shows the highest inhibition of 6.42 ± 0.004 compared to 35.63 ± 0.022% for gallic acid. The better antioxidant activity of gallic acid over the seed oil was further con-firmed from the IC50 value calculated from Figure 2c, which shows IC50 values of 28.00 and 1.59 µg/ml for seed oil and gallic acid, respectively.
The lower the antioxidant value, the higher the antioxidant potential.
Table 5d shows the nitric oxide scavenging antioxidant assay of Luffa aegyptiaca seed oil and gallic acid. The seed oil shows improved antioxidant activity comparable to gallic acid, as determined by the improved % inhibition and IC50 value in Figure 2d.
The low IC50 value of 3.75 µg/ml by the seed oil is comparable to that of gallic acid (1.16 µg/ml), indicating the huge potential of the seed oil to inhibit nitric oxide-induced oxidation of corrosion inhibition materials.
The presence of antioxidants in corrosion inhibition materials can help prevent oxidation of the materials, thereby improving the life span of corrosion inhibitors. The results are presented in Tables 6a – 6d for the initial antioxidant assay to which the oil was subjected. The antioxidant potential of renewable biosurfactant made from Luffa aegyptiaca seed oil was compared to sodium lauryl sulfonate (SLS), a synthetic surfactant.
Table 6a gives the results of the ABTS Radical Antioxidant Potential L. aegyptiaca seed oil biosurfactant and positive control SLS. As shown in Table 6a and Figure 3a, the biosurfactant shows a much better ABTS scavenging ability than SLS. The inhibition potential was dose dependent, and the highest inhibition of 15.58 ± 0.011 % was obtained for the seed oil biosurfactant compared to 11.14 ± 0.002 % for SLS. The IC50 value of the biosurfactant was lower (11.53 µg/ml) than the value (21.64 µg/ml) obtained for SLS, indicating the superior ABTS scavenging potential of the prepared biosurfactant and its potential to be incurporated into corrosion inhibition materials.
The biosurfactant shows a consistent sup-erior antioxidant potential over SLS, a synthetic antioxidant across Tables 6b – 6c and Figures 3b – 3c, with lower IC50 values for the biosurfactant to that of SLS, 1.97 to 2.08 µg/ml for DPPH radical antioxidant assay, 34.21 to 91.63 µg/ml for FRAP assay, respectively.
However, SLS shows better nitric oxide antioxidant assay than the prepared biosurfactant with IC50 value of 1.74 to 5.44 µg/ml in Table 6d and Figure 3d. The antioxidant activities shown by sponge seed oil biosurfactants are comparable to reported values by Rinaldiet al. [23] for neem oil biosurfactants.
Conclusion
The physical and chemical properties of the sponge seed make it viable for use as an industrial source of fat and oil due to its high yield of 25.96 ± 0.47%. The low free fatty acid content may be accounted for by the low peroxide value of 3.95±0.14 meq kg-1, which indicates the presence of natural phytochemicals present in the oil preventing the oxidation and hydrolysis of the oil and hence the low free fatty acids. The low free fatty acids also show that the oil is of high quality and has a long shelf-life. The high iodine value of 165.07±0.85 I2100 g-1 of oil exhibited by the seed oil accounts for why the oil is liquid at room temperature. The oil is largely made of unsaturated fatty acids with a total degree of unsaturation of 70.23%, as obtained from GC-MS analysis.
The biosurfactant shows appreciable efficiency in a salt tolerance level of 190 ppm above the range of 120 – 180 ppm for hard water, as stipulated by the US Water Corp-oration. Control synthetic surfactant SLS shows a salt tolerance of 220 ppm.
The clear absence of a hydroxyl group (O-H) in the seed oil and carbonyl group (C=O) in diethanolamine, which were prominent in the biosurfactant at 1618.33 cm-1, indicate the formation of a biosurfactant. The prepared biosurfactant shows a lower emulsion stability of 87.50 ± 2.53 seconds to 394 ± 1.41 seconds but better foaming stability, comparable to SLS used as a control.
Antioxidant analysis shows that oil has moderate antioxidant potential when compared to gallic acid, but the biosurfactant shows better antioxidant potential against ABTS radicals, DPPH radicals, and FRAP than SLS. SLS shows better nitric oxide (NO) antioxidant activity than the biosurfactant. This implies that the biosurfactant replaces its synthetic counterparts and helps against environmental pollution. It also shows that oil and biosurfactants can be used as starting ingredients for making protective coatings such as alkyd resin, while biosurfactants can be used as additives to protect against oxidation attacks by reactive chemical species.
References
- Araújo HW, Andrade RF, Montero-Rodríguez D, Rubio-Ribeaux D, Da Silva CAA, Campos-Takaki GM. Sustainable biosurfactant produced by Serratia marcescens UCP 1549 and its suitability for agricultural and marine bioremediation applications. Microb. Cell Fact., 2019, 18(1), 1-13.
- Lima RA, Andrade RF, Rodríguez DM, Araújo HW, Santos VP, Campos-Takaki GM. Production and characterization of biosurfactant isolated from Candida glabrata using renewable substrates. Afr. J. Microbiol. Res., 2017, 11(6), 237-244.
- Marchant R, Banat IM. Biosurfactants: A sustainable replacement for chemical surfactants? Biotechnol. Lett., 2012, 34(9), 1597-1605.
- Montero-Rodríguez D, Andrade RF, Ribeiro DLR, Rubio-Ribeaux D, Lima RA, Araújo H, Campos-Takaki GM. Bioremediation of petroleum derivative using biosurfactant produced by Serratia marcescens UCP/WFCC 1549 in low-cost medium. Int. J. Curr. Microbiol. Appl. Sci., 2015, 4(7), 550-562.
- Masakorala K, Turner A, Brown MT. Toxicity of synthetic surfactants to the marine macroalga, Ulva lactuca. Water, Air, Soil Pollut., 2011, 218(1), 283-291.
- Johnson P, Pinfield VJ, Starov V, Trybala A. Effect of synthetic surfactants on the environment and the potential for substitution by biosurfactants. Adv. Colloid Interface Sci., 2020, 102340.
- Geetha S, Banat IM, Joshi SJ. Biosurfactants: Production and potential applications in microbial enhanced oil recovery (MEOR). Biocatal. Agric. Biotechnol., 2018, 14, 23-32.
- Atlas RM, Hazen TC. Oil biodegradation and bioremediation: A tale of the two worst spills in U.S. history. Environ. Sci. Technol., 2011, 45(16), 6709-6715.
- Nitschke M, Costa S. Biosurfactants in food industry. Trends Food Sci. Technol., 2007, 18(5), 252-259.
- Jahan R, Bodratti AM, Tsianou M, Alexandridis P. Biosurfactants, natural alternatives to synthetic surfactants: physicochemical properties and applications. Adv. Colloid Interface Sci., 2020, 275, 102061.
- Stephens J. Gourd Luffa – Luffa cylindrica, Luffa aegyptiaca and Luffa acutangula. J. Hortic. Sci. Univ. Fla., 2003, 3, 19-21.
- Balakrishnan N, Huria T. Protective effect of Luffa cylindrica L. fruit in paracetamol induced hepatotoxicity in rats. Int. J. Pharm. Biol. Arch., 2011, 2(6), 1761-1764.
- Partap S, Kumar A, Sharma NK, Jha K. Luffa cylindrica: An important medicinal plant. J. Nat. Prod. Plant Resour., 2012, 2(1), 127-134.
- Zubair MF, Atolani O, Ibrahim SO, Oguntoye OS, Abdulrahim HA, Oyegoke RA, Olatunji GA. Chemical and biological evaluations of potent antiseptic cosmetic products obtained from Momordica charantia seed oil. Sustainable Chem. Pharm., 2018, 9, 35-41.
- Adewuyi A, Oderinde RA, Rao B, Prasad R. Synthesis of alkanolamide: A nonionic surfactant from the oil of Gliricidia sepium. J. Surfactants Deterg., 2012, 15(1), 89-96.
- Jain RM, Mody K, Mishra A, Jha B. Physicochemical characterization of biosurfactant and its potential to remove oil from soil and cotton cloth. Carbohydr. Polym., 2012, 89(4), 1110-1116.
- Nishaa S, Vishnupriya M, Sasikumar J, Hephzibah PC, Gopalakrishnan V. Antioxidant activity of ethanolic extract of Maranta arundinacea L. tuberous rhizomes. Asian J. Pharm. Clin. Res., 2012, 5(4), 85-88.
- Atolani O, Areh E, Oguntoye O, Zubair M, Fabiyi O, Oyegoke R, Tarigha D, Adamu N, Adeyemi O, Kambizi L, Olatunji G. Chemical composition, antioxidant, anti-lipooxygenase, antimicrobial, anti-parasite and cytotoxic activities of Polyalthia longifolia seed oil. Med. Chem. Res., 2019, 28(4), 515-527.
- Dash P, Panda P, Ghosh G. Free radical scavenging activities and nutritional value of Lagenaria siceraria: A nutriment creeper. Iran. J. Sci. Technol., Trans. A: Sci., 2018, 42(4), 1743-1752.
- Gangwar M, Gautam MK, Sharma AK, Tripathi YB, Goel R, Nath G. Antioxidant capacity and radical scavenging effect of polyphenol rich Mallotus philippenensis fruit extract on human erythrocytes: An in vitro study. Sci. World J., 2014.
- Alemayhu A, Admassu S, Tesfaye B. Shelf-life prediction of edible cotton, peanut and soybean seed oils using an empirical model based on standard quality tests. Cogent Food Agric., 2019, 5(1), 1622482.
- Adaramola B, Onigbinde A, Shokunbi O. Physiochemical properties and antioxidant potential of Persea americana seed oil. Chem. Int., 2016, 2(3), 168-175.
- Rinaldi F, Hanieh PN, Longhi C, Carradori S, Secci D, Zengin G, Ammendolia MG, Mattia E, Del Favero E, Marianecci C, Carafa M. Neem oil nanoemulsions: Characterisation and antioxidant activity. J. Enzyme Inhib. Med. Chem., 2017, 32(1), 1265-1273.
|