|
|
|
The Chemist Volume 93 | Number 1 |

|
|
Synthesis of α-Methylene Cinnamic Acid Using Sodium Hydroxide as a Catalyst Under Microwave Irradiation
|
|
Abstract: A simple, convenient synthesis of α-methyl cinnamic acid derivatives had been achieved by the Knoevenagel-Doebner condensation between succinic anhydride and substituted benzaldehyde using sodium hydroxide as a base under microwave irradiation. The present protocol has merits like easy workup, high yield, and avoiding the use of toxic solvents.
Key Words: α-methyl cinnamic acids, Knoevenagel condensation, sodium hydroxide, microwave irradiation, succinic anhydride, aromatic aldehyde
|
|
|
Introduction
Cinnamic acids are a type of compound constructed through the phenylpropanoid backbone (C6-C3) isolated from plants and microorganisms, showing fascinating biological activities [1]. They have been produced in the biochemical path that yields lignin, the polymeric material that furnishes mechanical assistance to the plant cell wall [2]. Cinnamic acids are generated in the biosynthetic pathway leading to phenylpropanoids, coumarins, lignans, isoflavonoids, flavonoids, stilbenes, aurones, anthocyanins, spermidines, and tannins [3]. Cinnamic acid, a natural aromatic carboxylic acid, is an important chemical found in plants such as Cinnamomum cassia (Chinese Cinnamon) and Panax ginseng, fruits, whole grains, vegetables, and honey. The presence of an acrylic acid group substituted on the phenyl ring gives cinnamic either a cis or a trans configuration, with the latter being the most common of the two [4]. Some naturally occurring bioactive cinnamic acid derivatives are shown in Figure 1. The cinnamic acid derivatives have been shown to have various biological activities [11].
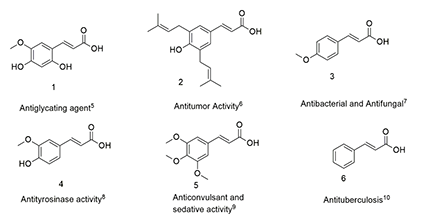 |
Cinnamic acids (α, β-unsaturated carboxylic acids) are reactive molecules due to the carboxylic acid and the polarized alkenyl moiety. Cinnamic acid derivatives are key starting materials for the synthesis of natural products, heterocyclic molecules, and biologically important molecules [12]. α-Methyl cinnamic acid moieties are the crucial structural units present in various biologically active molecules [13]. α-Methyl cinnamic acid derivatives are a valuable synthon for the synthesis of serine protease inhibitors. Due to the pharmaceutical importance of α-methyl cinnamic acids, many synthetic organic chemists are attracted to the synthesis of them. Very few methods have been reported in the literature for the synthesis of α-methyl cinnamic acid derivatives [14-22]. Some of these reported methods have shortcomings such as long reaction times, hazardous reaction conditions, use of toxic solvents. Hence, there is a need to develop a new synthetic methodology for such attractive molecules.
Microwave irradiation has gained much attraction in organic synthesis because it can be applied to activate numerous organic reactions efficiently. This technology furnishes a speedy way to obtain the desired products in high yield with a few minutes. Microwave irradiation was used in various organic transformations [23-30]. Here, we have studied the synthesis of α-methyl cinnamic acid starting from succinic anhydride and various substituted benzaldehyde. Sodium hydroxide was used as a base reagent under microwave irradiation.
Results and Discussion
The condensation of benzaldehyde and succinic anhydride with a base was selected as a model reaction (Figure 2). Initially, the various metal hydroxide and carbonates, such as sodium carbonate, potassium carbonate, calcium carbonate, magnesium carbonate, sodium hydroxide, potassium hydroxide, lithium hydroxide, sodium hydrogen carbonate, strontium carbonate, and barium carbonate, act as a catalyst for the condensation reaction at room temperature and microwave conditions.
Results are summarized in Table 1. The model reaction is performed in a 50-mL beaker using 5 mmol of benzaldehyde, 5 mmol of succinic anhydride and catalyst in microwave ovens. From Table 1, it was observed that NaOH was found to be an efficient catalyst for the synthesis of α-methyl cinnamic acid with 95% yield.
Similarly, succinic anhydride (5 mmol) and benzaldehyde (5 mmol) were selected as the model substrate to optimize the amount of NaOH. The catalyst loading has been optimized by increasing the amount of NaOH from 10 mol % to 50 mol % for a 5 mmol scale reaction. When the reaction was carried out in the absence of a catalyst, the product formed in minor quantities and the time required to form the product was long (Table 2, entry 1). The yield has increased with the increase in catalyst amount (Table 2, entry 2-5). From the table, it was observed that a 50 mol % catalyst is sufficient to obtain the best yield in a short reaction time.
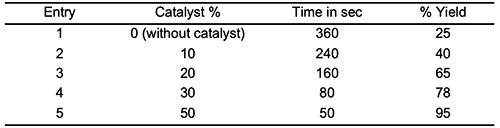 |
|
To study the effect of power watt on the reaction, the model reaction of 1 mmol of benzaldehyde, 1 mmol of benzaldehyde, and 50 mol % of sodium hydroxide was used. The reaction was irradiated at 100W, 200W, 300W, 400W, 600W, 800W and 1200W. The results of the study are tabulated in Table 3 and the reaction well proceeded at 600W. Hence, all the reactions were performed at 600W in the microwave oven.
With these optimized reaction conditions in hand, we have studied the convenience of the method and it has been well evaluated using a variety of substituted aryl aldehydes for the synthesis of a series of compounds with this simple approach (Figure 3).
The results are summarized in Table 4. The nature and position of the functional groups on the phenyl ring affected the reaction time and yields of the product. The results indicated that aromatic ring bearing electron-donating groups such as -OCH3, -CH3, -NMe2 and withdrawing groups like nitro were afforded high yields of product. The hydroxy substituted aldehydes did not yield the respective cinnamic acid due to reaction of sodium hydroxide with phenolic -OH group, rather with succinic anhydride and decrease in electrophilicity of aldehyde carbonyl carbon by phenoxide ion.
Conclusion
Here, we have reported the efficient synthesis of α-methyl cinnamic acid via a condensation reaction between an aromatic aldehyde and succinic anhydride using aqueous sodium hydroxide solution under microwave irradiation. This novel approach has been used in the preparation of various ɑ-methyl cinnamic acids at good to high yields (up to 95%). The main advantage of the present protocol is the high yield, operational simplicity, easy workup, easily available, and inexpensive catalyst. The present protocol did not proceed with the free -OH group and indole due to the acidic character of the phenolic OH and indole.
Experimental
Melting points were measured using the open capillary method and are uncorrected. IR spectra were recorded on Alpha T BRUKER model. 1H NMR and 13C NMR spectra were recorded at ambient temperature on a BRUKER AVANCE DRX-400 MHz spectrophotometer using CDCl3 or DMSO-d6 as the solvent and TMS as an internal standard. The purity of newly synthesized compounds and the development of reaction were monitored by thin layer chromatography (TLC) on Merck pre-coated silica gel 60 F254 aluminium sheets, visualized by UV light.
A mixture of aromatic aldehyde (5 mmol), succinic anhydride (5 mmol) and sodium hydroxide (2.5 mmol) was placed in a 50-mL borosil beaker. The reaction mixture was mixed properly with a glass rod. The mixture was irradiated in a microwave oven at 600 W for an appropriate time (monitored by TLC, Table 4). On cooling, the reaction mass was acidified with dil. HCl; the product was precipitated out from the reaction mixture. The product was isolated by filtration followed by washing with water. The isolated product was pure enough and further purified by crystallization from ethanol. All products were known, and their structure has confirmed by spectral data matching with authentic samples.
References
- Zolfaghari B, Yazdiniapour Z, Sadeghi M, Akbari M, Troiano R, Lanzotti V. Phytochem. Anal., 2021, 32(1), 84-90.
- Inokuchi R, Takaichi H, Kawano T. Environ. Control Biol., 2016, 54(1), 57-64.
- Vogt T. Phenylpropanoid biosynthesis. Mol. Plant, 2010, 3, 2-20.
- Ruwizhi N, Aderibigbe BA. Int. J. Mol. Sci., 2020, 21, 5712.
- Muhammad N, Saeed M, Adhikari A, Khan KM, Khan H. J. Enzyme Inhib. Med. Chem., 2013, 28, 997-1001.
- Del P, Baltas M, Bedos-Belval F. Curr. Med. Chem., 2011, 18, 1672-1703.
- Nakazono Y, Watanabe Y, Hashinaga F, Tadera K. J. Biol. Sci., 2006, 6, 135-139.
- Lee H.-S. J. Agric. Food Chem., 2002, 50, 1400-1403.
- Zhao Z, Song H, Xie J, Liu T, Zhao X, Chen X, He X, Wu S, Zhang Y, Zheng X. Eur. J. Med. Chem., 2019, 173, 213-227.
- De P, B-Belvala F, V-Bacquéa C, Balts M. Curr. Org. Chem., 2012, 16, 747-768.
- Asif M, Imran M. Prog. Chem. Biochem. Res., 2019, 2, 12-210.
- Chen L, Zhang L, Yan G, Huang D. Asian J. Org. Chem., 2020, 9, 842-862.
- Basavaiah D, Krishnamacharyulu M, Hyma RS, Sarma PKS, Kumaragurubaran N. J. Org. Chem., 1999, 64, 1197-1200.
- Still WC, Gennari C. Tetrahedron Lett., 1983, 24, 4405-4408.
- Teulade M-P, Savignac P, About-jaudet E, Collignon N. Synth. Commun., 1989, 19, 71-81.
- Rossi R, Carpit A, Cossi P. Tetrahedron, 1992, 48, 8801-8824.
- Myrboh B, Ila H, Junjappa H. J. Org. Chem., 1983, 48, 5327-5332.
- Zargarian D, Alper H. Organometallics, 1993, 12, 712-724.
- Beesu M, Periasamy M. J. Organomet. Chem., 2012, 705, 30-33.
- Song C-X, Chen P, Tang Y. RSC Adv., 2017, 7, 11233-11243.
- Carpino LA. J. Am. Chem. Soc., 1958, 80, 601-604.
- Wehrmeister HL. J. Org. Chem, 1962, 27, 4418-4420.
- Ahmadi M, Moradi L, Sadeghzadeh M. J. Mol. Struct., 2021, 1235, 130183.
- Dev D, Kalita T, Mondal T, Mandal B. Adv. Synth. Catal., 2021, 363, 1427-1435.
- Singh J, Nickel GA, Cai Y, Jones DD, Nelson TJ, Small JE, Castle SL. Org. Lett., 2021, 23, 3970-3974.
- Wolf L, Mayer JCP, Quoos N, Sauer AC, Schwab RS, Rodrigues OED, Dornelles L. Tetrahedron, 2021, 91, 132222.
- Abirami M, Selvi ST, Nadaraj V. Lett. Org. Chem., 2021, 18, 156-159.
- Kulkarni P. J. Indian Chem. Soc., 2021, 98, 100013.
- Shahari MSB, Junaid A, Tiekink ERT, Dolzhenko AV. Synthesis, 2021, 53, 2457-2468.
- Kadagathur M, Sigalapalli DK, Patra S, Tangellamudi ND. Synth. Commun., 2021, 51, 2213-2224.
- Gensler WJ, Berman E. J. Am. Chem. Soc., 1958, 80, 494-4954
- Myrboh B, Asokan CV, Ila H, Junjappa H. Synthesis, 1984, 50-51.
|