|
|
|
|
The Chemist Volume 95 | Number 1 |

|
|
Chemical Characterization, Cytotoxicity, Anti-inflammatory, Antioxidant, Antimicrobial and Anti-toxoplasmosis of Chrysophyllum Albidum Seed Oil
|
|
Abstract: Chrysophyllum albidum, an important tropical fruit with seeds, was investigated
for its chemical composition, cytotoxic free radical scavenging, anti-inflammatory,
antimicrobial (on clinical isolates) and antiparasitic properties for prospective application in
the cosmeceuticals and food sectors. The physicochemical results of the C. albidum oil were
179.52±2.80 mg KOH/g saponification value, 3.93±0.56 mg KOH/g acid value, 1.58±0.11%
free fatty acid and 39.34±2.54 g I2/100 g iodine value, suggesting the suitability of the oil for
industrial purposes, such as in skin care production. C. albidum seeds contained linoleic acid
(33.12%), oleic acid (31.81%), palmitic acid (17.68%) and stearic acid (5.30%) as the major
fatty acids. The IC50 value estimated for the anti-lipooxygenase potential of the oil
(0.56±0.09 µg/mL) was close to that obtained for the standard indomethacin (0.53± 0.07
µg/mL). The IC50 obtained for the DPPH (4.74±0.83 µg/mL) and ABTS (0.03±0.11 µg/mL)
of the oil was significant but relatively low compared to that of ascorbic acid (0.34±0.04 and
0.54±0.04 µg/mL) used as standards for the DPPH and ABTS free radical scavenging
activities, respectively. The oil had significant activities against all the clinical isolates, with
A. niger showing a significant MIC of 12.5 mg/mL. The seed oil of C. albidum possesses
numerous biological activities that can be harnessed. The presence of essential fatty acids is
partly suggestive of the observed bioactivities. Hence, the oil of C. albidum seeds with
significant health benefits holds potential for possible incorporation into food,
pharmaceutical and cosmeceutical products.
Key Words: Chrysophyllum albidum, oleic acid, lipoxygenase, antioxidant, cytotoxicity,
antiparasite
|
|
|
Introduction
Seeds are the major sources of natural oil, known as fixed oil. Fixed oil belongs to a class of hydrolysable lipids that dissolve completely in nonpolar organic solvents such as n-hexane but are insoluble in aqueous media. The major component of fixed oil is triglycerides, making up 85% of the total percentage of its constituents. The minor components of fixed oil are mono-/diglycerides; tocopherol (vitamin E), which is often responsible for the antioxidant property of the oil; chlorophyll/carotenoids, which are responsible for the colouration of the oil; and other minor components, such as steroids, triterpenes, and phospholipids, which are responsible for other biological activities [1,2].
Fixed oil is classified into nondrying, semidrying and drying based on the iodine index, which refers to the quantity in grams of iodine consumed per unit of the oil. This classification helps to determine the potential application of oils. Nondrying oil has an iodine value below 100 gI2/100 g and is suitable for consumption (vegetable oil) and industrial purposes, such as in the production of soaps. Semidrying oil has iodine values greater than 100 gI2/100 g and less than 250 gI2/100 g, and this oil is suitable for the production of lubricant and brake fuel in the automobile industry. Drying oil has an iodine value greater than 260 gI2/100 g. These types are more applicable for the industrial production of paint, ink, etc. [3,4].
Chrysophyllum albidum, of the family Sapotaceae [5] and is a common plant throughout the Asian and African tropics. The ripe fruit is ovoid with a pointed apex and bears two to eight seeds. The fruit contains seeds with hard lustrous brownish seed coats. The fruits are produced between January and March annually [6]. Phytochemically, stems and seeds reportedly contain secondary metabolites, including phenolics, flavonoids, glycosides and alkaloids [7]. Methanol extracts of C. albidum cotyledon seeds reportedly contain eleagnine, tetrahydro-2-methylharman and skatole [6]. Extracts from the root and seed are used as wound healing agents [7].
The plantreportedly possesses good free radical scavenging activities [5,8]. In most regions where the plant thrives, the numerous seeds of the plant are often discarded and grossly underutilized. To establish the pharmacological potential of bioactivities, this work investigated the chemical compositions, radical scavenging, inhibition of inflammation progression, and antiparasitic and cytotoxic activities of highly underutilized C. albidum seeds for possible application in the food or cosmetic/pharmaceutical industries.
Trolox, ascorbic acid, 1,1-diphenyl-2-picryhydrazyl (DPPH), 2,2-azino-bis-(3-ethylbenzothiazoline-6-sulfonic acid (ABTS), antioxidant and anti-inflammatory assay kits, which were high purity grades, were obtained from Santa Cruz Biotechnology, USA. Indomethacin, staurosporine, and fatty acid standards were obtained from Sigma Reagent Company.
C. albidum fruits from which the seeds were collected were obtained at Ilorin, Nigeria, in December 2016 during the fruiting season. The plant material with voucher number UILH/002/1216 was subjected to standard identification and valid authentication at the Herbarium of the University of Ilorin, Ilorin, Nigeria. The seeds were subsequently subjected to drying under the shade, followed by deshelling and pulverization.
The C. albidum seed oil was obtained via Soxhlet extraction using hexane at 60°C followed by extract concentration at low temperature using an automated recirculation rotary evaporator [9]. The hexane extract was subjected to transmethylation and analyzed using gas chromatography–mass spectrometry. For the antioxidant potential, standard assays of 2,2-azino-bis-(3-ethylbenzothiazoline-6-sulfonic acid) and 2,2-diphenyl-1-picrylhydrazyl were adopted. To evaluate the antibacterial and antifungal activities of the oil, isolates that included Salmonella typhi, Bacillus subtilis, Klebsiella pneamananae, Staphylococcus aureus, Pseudomonas aeruginosa, Escherichia coli andfungi, including Aspergillus niger, Rhizopus stolonifer, Penicillium notatum and Candida albicans, were used. The anti-inflammatory activity was measured using a lipooxygenase assay, while the cytotoxicity potential was measured using mammalian cell assays, with indomethacin and staurosporine used as standards. The antiparasitic activity (anti-toxoplasmosis) was also evaluated.
Initially, the concentrated seed oil (100 mg) was constituted in hexane (10 mL), while an aliquot was further reconstituted in hexane to afford a more dilute concentration as desired following a standard procedure. An internal standard, heptadecanoic acid (C -17:0) (100 µL of 100 ppm) and 1 mL of 2.5% (v/v) methanolic sulfuric acid were added to the dilution. The mixture was incubated in an oven and maintained at 80°C for 1 hour followed by cooling to ambient temperature (25°C). NaCl solution (1.5 mL) of 20% (w/v) was added to the mixture, vigorously shaken and separated by centrifugation. The upper hexane layer (containing the methylated products) was separated, concentrated and subjected to elution in standardized gas chromatography equipment. Next, the amount of trans-esterifiable oil was determined and noted.
To profile the fatty acid content of the trans-esterified seed oil, the oil was subjected to GC–MS analysis on an Agilent Gas Chromatograph (6890N) equipped with an autosampler. The elution was performed on a nonpolar column of 30 m by 0.25 x 0.25 µm using helium as the carrier gas. The injection volume, flow rate, injection temperature and split ratio were 1 µL, 1 mL/min, 250°C and 5:1, respectively. The heating temperature was adjusted to 100°C (5 min), then 180°C 5°C per minute (kept for five minutes) and finally raised to 330°C at 8°C per minute (stabilized for five minutes). A coupled mass analyser (5975B, Agilent Technologies) with electron impact mode was adopted to generate the fragmentations, which were used to identify the fatty acid ester in addition to the standard used. The mass spectrometer operating at 70 eV (electron impact mode) had a scan range of 35 to 500 m/z.
The fresh seed oil and the trans-esterified seed oil were both subjected to FT-IR analysis to identify functional group transformations using an infrared spectrometer (Nicolet iS5 FT-IR) by preparing KBr pellets.
The absorption maximum was determined on an ultraviolet–visible spectrophotometer (Beckman, UK), while a multiscan spectrophotometer [Spectra Max (Plus) US] was used to measure the absorbance measurements for the assays.
The physicochemical parameters, including iodine value (IV), saponification values (SV), free fatty acids (FFA) and acid value (AV), were determined following a standard protocol [4,10,11].
The in vitro DPPH assay to evaluate the antioxidant potential of C. albidum seed oil was carried out following a standard procedure [12,13]. Using α-tocopherol as a standard reference, the concentrations of the samples were varied (10 – 500 µg/mL). To each sample concentration (2.4 mL), 0.8 mL DPPH (0.1 mM) solution in methanol was added, thoroughly homogenized and kept in the dark at ambient temperature (ten minutes) before termination. The absorbance of the mixture was measured at 517 nm on a scan absorbance reader (Thermo Spectronic, UK).
In this assay, nordihydroguaiaretic acid and rutin served as reference inhibitors of lipoxygenase following a standard method [15]. In the absence of inhibitors, the lipoxygenase solution (50 µL) was mixed with sodium linoleate (2.0 mL) at 100 µM. An aliquot (30 µL) of the inhibitor dissolved in dimethylsulfoxide (DMSO) was added to make a solution with 100 ppm. The experiment was carried out in triplicate. The absorbance value was measured on an absorbance spectrophotometer, and the extent of inhibition was determined.
Antibacterial and antifungal activities were examined by the disc agar diffusion method as previously described [16]. For the antibacterial assay, inoculates of clinical isolates of McFarland standards (Escherichia coli, Pseudomonas aeruginosa, Staphylococcus aureus, Bacillus subtilis, Salmonella typhi and Klebsiella pneumoniae) were prepared. Thereafter, the plates were impregnated with each oil solution at 62.5 to 500 mg/mL and were prepared by diluting the oil with distilled water. Thereafter, the air-dried impregnated discs were positioned on the surface of the agar plates.
For the antifungal assay, four clinical isolates (Penicillium notatum, Candida albicans, Rhizopus stolonifera and Aspergillus niger) were inoculated into mycological peptone. After 1 h of incubation, the inoculant was used to swab Sabouraud’s dextrose agar. Incubation was performed at 37°C for 24 h and 48 h for bacterial and fungal strains, respectively. Reference drugs for antibacterial and antifungal activities included gentamycin and tioconazole, respectively, while discs with water only served as controls. To determine the antimicrobial activity of the oil, the diameter (millimeters) of the clear regions of inhibition were measured. The observable clear inhibition regions were taken as evidence of antimicrobial action and vice versa.
The MIC of the sample was determined using the disc diffusion method [16]. Briefly, the reconstituted sample in agar medium was applied to the dish. After incubating for 24 and 48 h at 37°C and 27°C for bacteria and fungi, respectively, the clear inhibition regions were determined in mm. While sterile medium was used as a control, the MIC was taken as the sample with the lowest concentration causing complete retardation of microbial growth.
The cytotoxicity assay using human fibroblast forekin (HFF ATCC®) cells was performed following a procedure previously reported [17,18], as staurosporine-treated cells served as a positive control drug. The antiparasite (in vitro anti-Toxoplasma gondii) assay on monolayers of HFF cells was performed using a luciferase-active T. gondii RH strain 2F, following a standard procedure we reported previously [19-22]. All assays were performed in triplicate independently in 96-well plates (Nunc; Fisher Scientific, Pittsburgh, PA, USA) unless otherwise stated.
Following the determination of the antiparasitic potential of the seed oil, the mode of antiparasitic action was also determined via the evaluation of the culpability of reactive oxygen species (ROS) by including Trolox, an antioxidant, in the screening assay. Parasite growth inhibition was determined as described above. Thereafter, the level of ROS in cells was determined on HFF monolayers as previously described [17,23].
Data obtained from the assays were subjected to statistical evaluation using one-way ANOVA (GraphPad Prism Software Inc., San Diego, CA), as the results are reported as the mean of three replicates ± standard error of the mean (SEM). The IC50, the concentration that induced 50% inhibition in parasite and/or mammalian host cell growth, was estimated using a nonlinear regression analysis on a dose–response curve in GraphPad Prism 5.
Results and Discussion
The C. albidum seed afforded (via Soxhlet extraction) an oil yield of 2% of the total weight of the seed material, of which 95% was trans-esterifiable. The physicochemical parameters of the oil determined following standard procedures are shown (Table 1).
The oil has a saponification value of 179.52±2.80 mg KOH/g (Table 1). The high saponification values exhibited by this oil suggest that the oil will offer promising benefits in cosmetic and personal care products [24,25]. A high concentration of short-chain fatty acids has been implicated in fats and oils with high saponification value [26].
The seed oil has an acid value of 3.93±0.56 mg/KOH/g (Table 1). Low acid values in oil correspond to relative stability with attendant resistance to rancidity and peroxidation. However, a low acid value is also associated with the presence of phenolics and antioxidants [4,27]. C. albidum had a relatively low free fatty acid value of 1.58±0.11 (Table 1).
To estimate the level of unsaturation in fatty acids, the iodine value was used. This is achieved by reacting fatty acids with iodine compounds. Lower iodine values correspond to harder texture and reduced propensity for oxidative rancidity. The iodine value and saponification value are complementary. The C. albidum seed oil had an IV of 39.34±2.54, confirming it as a nondrying oil.
Characterization
The extent of the transmethylation of the oils was established using infrared analysis, and the important peaks are as indicated (Table 2).
The diminished O-H vibration at 3439 cm-1 confirms that trans-esterification was successful. The vibrations at 1745 and 1742 cm-1 in the crude and trans-esterified oils, respectively, are typical of carboxylic esters and correspond to carbonyl stretching [28].
The GC–MS result of the oil is depicted in Table 4.
The C. albidum trans-esterified seed oil subjected to GC–MS analysis revealed prominent fatty acids, which included linoleic acid (33.12%), oleic acid (31.81%), palmitic acid (17.68%) and stearic acid (5.30%) (Table 4). Meanwhile, 26.08% of the fatty acid proportion in the oil of C. albidum was saturated.
Linolenic acid (omega-3) and linoleic acid (omega-6) are polyunsaturated fatty acids termed essential fatty acids that are required by humans for nutritional purposes but cannot be synthesized by the body. Unlike omega-3 and omega-6, oleic acid, an omega-9 fatty acid, and palmitic acid are important fatty acids found in plant seeds and are very useful in industries for various purposes, such as in paint production and cosmetic formulation. Linoleic acid is also often applied in cosmetic production, as it is reputable for its ability to moisturize the skin while contributing to wound healing and other dermal conditions. Linoleic acid- and linolenic acid-rich oils have been identified as good natural sources of anti-inflammatory agents for acne treatment [3,29]. In addition, palmitic and linoleic acid deficiency in the human diet manifests in physiological forms such as nail cracking, hair loss, skin dryness and scaling. Linoleic acid is a widely applied fatty acid in cosmetics because it moisturizes the skin, aids in the skin healing process, and reduces sunburns and acne treatment [29,30]. Oleic and palmitic acids reportedly permeate the skin to reach sensitive human parts. Furthermore, other micronutrients present in seed oils may prevent the risk of cardiovascular diseases by reducing cholesterolemia and oxidative stress. These micronutrients include phytosterols and various antioxidants, such as polyphenols, tocopherols and coenzymes. Seed oils are widely used in folkloric medicine worldwide, as they serve as potential sources of phytochemicals with antibacterial, antifungal and antiparasitic properties. Therefore, the chemical composition of the oils of C. albidum suggests that it may be applicable in food and cosmeceutical preparation, as it may also find application in drug and supplement production if well harnessed.
Lipoxygenases (LOXs) are important enzymes that convert arachidonic acid, linoleic acid and other polyunsaturated fatty acids into bioactive metabolites that are responsible for inflammatory and immune responses. These enzymes are key in the biosynthesis of leukotrienes and play vital roles in several inflammation-related diseases, such as cancer, allergic reactions, asthma, colitis ulcerosa, rheumatoid arthritis, and psoriasis [31-34]. The inhibition of leukotriene biosynthesis via the LOX pathway with natural products may have occurred by potential therapeutic means. The anti-LOX activity (anti-inflammatory activity) of the seed oil of C. albidum was determined and compared with a standard drug, indomethacin, following a standard procedure already described. A dose response activity comparable to that of the standard drug was obtained (Table 5 and Figure 1).
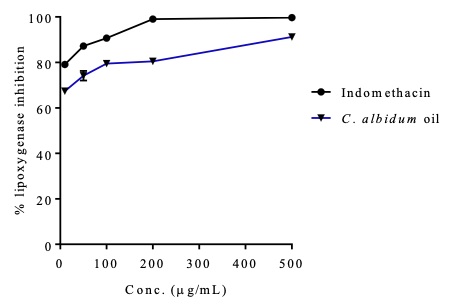 |
The results in Table 5 show that the inhibition of the oil is dose dependent, and the corresponding IC50 values of both the oil and indomethacin were 0.56 ± 0.09 and 0.53 ± 0.07 µg/mL, respectively. Seed oil exhibited potent LOX inhibitory activity and is suggested to possess anti-inflammatory activity.
For the evaluation of the free radical scavenging capacity of the C. albidum seed oil, two antioxidant assays (DPPH and ABTS) were adopted. Precisely, the oil showed a DPPH dose–response scavenging action (IC50 = 4.74±0.83 µg/mL), which was lower than the activity observed in the standard, ascorbic acid (IC50 = 0.34±0.04 µg/mL), as depicted in Table 6 and Figure 2.
The ability of the oil to scavenge ABTS radicals was evaluated using a standard procedure. The oil did not exhibit concentration-dependent properties (Table 7, Figure 3) but was significant with a value (IC50 = 0.03±0.11 µg/mL) when compared with ascorbic acid (IC50 = 0.54±0.04 µg/mL).
From the antioxidant assay results (Figures 2 and 3), the oil exhibited some potential to scavenge DPPH and ABTS radicals.
The oil exhibited appreciable inhibition (200 mg/mL) of the growth of all the test organisms (Table 8 and Figure 4). E. coli, P. aeruginosa and A. niger at 200 mg/mL exhibited the highest antimicrobial sensitivity (17±1 mm) to the C. albidum seed oil, while R. stolonifer exhibited the lowest sensitivity (13±1 mm).
The lowest MIC was recorded against A. niger at a concentration of 12.5 mg/mL and an inhibition diameter of 10 mm. The C. albidum seed oil had significant activity, which was lower than the activity recorded for gentamicin. Various natural products have shown good antimicrobial activities with potential for further development [11,35-39].
The in vitro cytotoxic potential of C. albidum oil on HFF mammalian cells was determined. The cellular toxicity (Table 9) was lowest at 250 µg/mL, with cell viability (128.64%), while at 1000 µg/mL, cell viability was 0.03%. As shown in Figure 5, C. albidum revealed dose-dependent cytotoxic activities against HFF cells. Dégbé et al. [40] demonstrated the low cytotoxic effect of an ethanolic extract of Vernonia amygdalina on mammalian cells as well as moderate antioxidant properties.
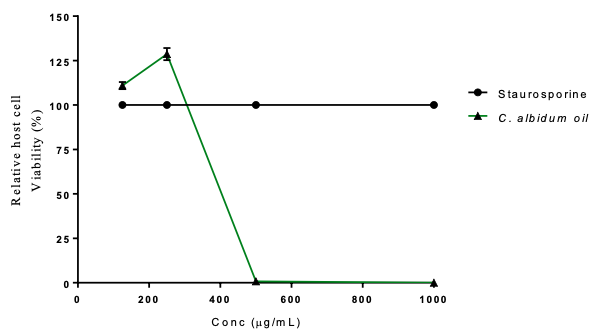 |
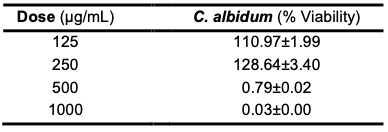 |
T. gondii, a common protozoan parasitic agent responsible for toxoplasmosis, is known to possess the capability to infect nearly all warm-blooded animals [17,18]. In more than the 1 billion human populations that it infects, it often has lethal outcomes in pregnant women, although it is asymptomatic in other healthy individuals. T. gondii human infection occurs through the consumption of uncooked or improperly boiled infected meat [41,42]. In this study, C. albidum exhibited concentration-dependent activities against T. gondii parasite growth (Table 10). Precisely at 125 and 250 µg/mL, the oil had 97.89% and 113.39% parasite growth, respectively (Figure 6).
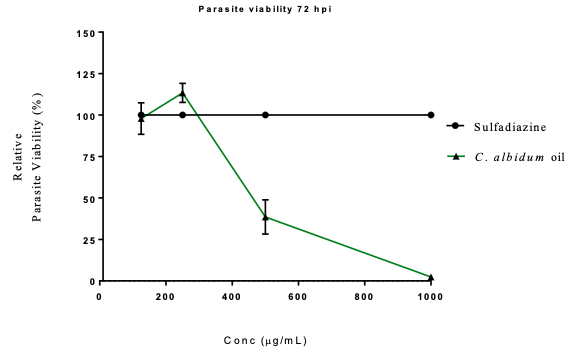 |
Conversely, at 500 and 1000 µg/mL, the oil exhibited intense inhibition of T. gondii parasite growth. The in vitro antiparasitic action exhibited is attributable to the quality of the phytochemicals constituting the seed oils. In previous studies, the ethanol extract of Vernonia amygdalina reportedly inhibited T. gondii activity [40]. Similarly, P. betle has been shown to be a potential candidate in the treatment of toxoplasmosis by inhibiting parasite growth in vitro in HFF cells and promoting survival in an experimental model [43]; the growth inhibitory properties of Lepidium perfoliatum and L. sativum against T. gondii tachyzoites have also been reported [44].
Following the establishment of the parasite inhibition potential of the oil, we sought to determine the likely mode of antiparasitic action. Therefore, we investigated the culpability of ROS in the inhibition potential by the inclusion of Trolox, an antioxidant at a concentration of 100 µM, in the screening.
As seen in Figure 7a-b, the Trolox inclusion in the screening assay induced a significantreduction in the inhibition potential. The results obtained for ROS analysis revealed that the C. albidum oil did not boost the ROS secretion both at the inclusion and exclusion of T. gondii infection (Figure 8a-b). In contrast, C. albidum oil impaired the fluorescing capacity of the cellular mitochondrial membrane both when T. gondii infection was absent (Figure 8c) and present (Figure 8d). The emerging observation is suspected to be a result of T. gondii infection leading to the disruption of the physiological integrity of cells.
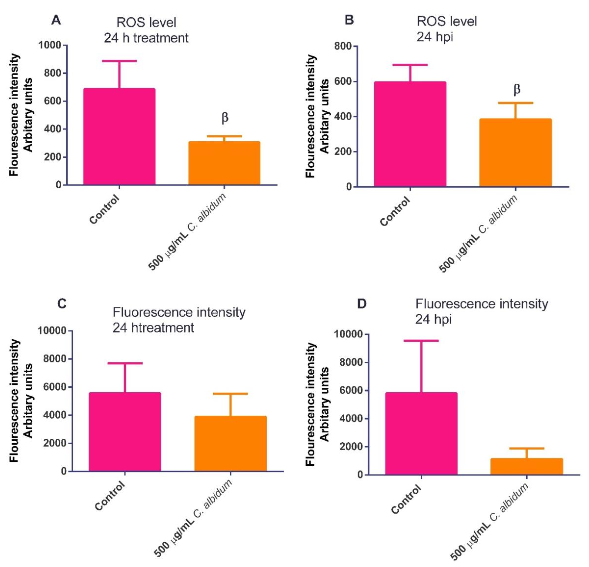 |
To determine whether the inhibition of T. gondii growth by C. albidum oil was a result of specific or general toxicity, the selectivity index (SI) of the sample was estimated by calculating the ratio of the cytotoxicity (IC50) in mammalian cells to the antiparasite activity (EC50). As shown in Table 11, the SI (<1) of C. albidum was found to be lower than that of the standard drug sulfadiazine (<4). While the C. albidum oil showed good inhibition potential against T. gondii growth, it could not exhibit satisfactory selectivity toward the parasite versus the host cells. The results obtained in this study revealed that the inhibitory activity of the C. albidum seed oil was due to general cellular toxicity or by an underlying antiparasitic mechanism beyond the scope of the present study.
Conclusions
The anti-LOX activity (anti-inflammatory activity) of the seed oil of C. albidum has a dose response activity comparable to that of the standard drug (indomethacin). The DPPH dose–response scavenging action of the oil (IC50 = 4.74±0.83 µg/mL) is lower than the activity observed in the standard, ascorbic acid (IC50 = 0.34±0.04 µg/mL), while in ABTS analysis the oil extract shows a significant activity higher than the ascorbic acid. The different in the antioxidant activities is due to different mechanism of action of the assay.
In this study, we showed that C. albidum seed oil contained good amounts of industrially useful fatty acids, of which oleic acid, linoleic acid and palmitic acid are major. The oil exhibited several biological properties, including anti-lipooxygenase, antioxidant, anti-inflammatory, anti-toxoplasmosis, cytotoxic and antimicrobial potential. Of important attention are the antimicrobial, anti-lipooxygenase and anti-toxoplasmosis potential, which hold prospects for further development. The exploration of many underutilized tropical seed oils in recent times has opened a new channel of research for further investigation. C. albidum seed oil, which is often discarded, holds promise for industrial utilization. Considering these results together, the biological activities shown by the grossly underutilized seed oil of C. albidum could be effectively exploited in food processing, nutraceutical and cosmeceutical development. For example, if the oil extract of C. albidum is incorporated into personal care products applied topically, it may prevent or clear skin infections and inflammation-related conditions.
Acknowledgments
The authors are grateful to The World Academy of Sciences (TWAS) for some financial support via research grant: 15-244 RG/CHE/AF/AC_G – FR3240287031.
References
- Aluyor EO, Ozigagu CE, Oboh OI, Aluyor P. Chromatographic analysis of vegetable oils: A review. Sci. Res. Essays, 2009, 4, 191-197.
- Mohammad A. General chemistry, composition, identification and qualitative tests of fats or oils. J. Pharm. Res. Opin., 2011, 2, 52-64.
- Vermaak I, Kamatou GPP, Komane-Mofokeng B, Viljoen AM, Beckett K. African seed oils of commercial importance - Cosmetic applications. S. Afr. J. Bot., 2011, 77, 920-933.
- Atolani O, Olabiyi ET, Issa AA, Azeez HT, Onoja EG, Ibrahim SO, Zubair MF, Oguntoye OS, Olatunji GA. Green synthesis and characterization of natural antiseptic soaps from the oils of underutilized tropical seed. Sustain. Chem. Pharm., 2016, 4, 32-39.
- Adebayo AH, Abolaji AO, Kela R, Ayepola OO, Olorunfemi TB, Taiwo OS. Antioxidant activities of the leaves of Chrysophyllum albidum G. Pak. J. Pharm. Sci., 2011, 24, 545-551.
- Amusa NA, Ashaye OA, Oladapo MO. Biodeterioration of the African star apple (Chrysophylum albidum) in storage and the effect on its food value. Afr. J. Agric., 2013, 1, 11-13.
- Okoli BJ, Okere OS. Antimicrobial activity of the phytochemical constituents of Chrysophyllum albidum G. Don_Holl. (African star apple) plant. J. Res. Natl. Dev., 2010, 8, 1-5.
- Idowu TO, Iwalewa EO, Aderogba MA, Akinpelu BA, Ogundaini AO. Antinociceptive, Anti-inflammatory and antioxidant activities of eleagnine: An alkaloid isolated from Chrysophyllum abidum seed cotyledons. J. Biol. Sci., 2006, 6, 1029-1034.
- Atolani O, Areh ET, Oguntoye OS, Zubair MF, Fabiyi OA, Oyegoke RA, Tarigha DE, Adamu N, Adeyemi OS, Kambizi L, Olatunji GA. Chemical characterization, antioxidant, cytotoxicity, anti-Toxoplasma gondii and antimicrobial potentials of the Citrus sinensis seed oil for sustainable cosmeceutical production. Heliyon, 2020, 6, 2, e03399.
- Atolani O, Adeosun CB, Adeniyi O, Kayode O. Antioxidant and direct transmethylation of lipids from Sabal causiarum seed. J. Appl. Pharm. Sci., 2015, 5(3), 24-28.
- Atolani O, Areh ET, Oguntoye OS, Zubair MF, Fabiyi OA, Oyegoke RA, Tarigha DE, Adamu N, Adeyemi OS, Kambizi L, Olatunji GA. Chemical composition, antioxidant, anti-lipooxygenase, antimicrobial, anti-parasite and cytotoxicity of Polyalthia longifolia seed oil. Med. Chem. Res., 2019, 22, 12.
- Schmeda-Hirschmann G, Rodriguez JA, Theoduloz C, Astudillo SL, Feresin GE, Tapia A. Free-radical scavengers and antioxidants from Peumus boldus Mol. (“Boldo”). Free Radical Res., 2003, 37, 447-452.
- Valyova M, Stoyanov S, Markovska Y, Ganeva Y. Evaluation of in vitro antioxidant activity and free radical scavenging potential of variety of Tagetes erecta L. flowers growing in Bulgaria. Int. J. Appl. Res. Nat. Prod., 2012, 5, 19-25.
- Re R, Pellegrini N, Proteggente A, Pannala A, Yang M, Rice-Evans C. Antioxidant activity applying an improved ABTS radical cation decolorization assay. Free Radical Biol. Med., 1999, 26, 1231-1237.
- Njenga EW, Viljoen AM. In vitro 5-lipoxygenase inhibition and anti-oxidant activity of Eriocephalus L. (Asteraceae) species. S. Afr. J. Bot., 2006, 72, 637-641.
- Atolani O, Oladoye SO, Adeyemi OS, Olatunji GA. In vitro antiproliferative and antimicrobial evaluation of Kigelia pinnata. Environ. Exp. Biol., 2014, 12, 29-32.
- Adeyemi OS, Murata Y, Sugi T, Kato K. Inorganic nanoparticles kill Toxoplasma gondii via changes in redox status and mitochondrial membrane potential. Int. J. Nanomed., 2017a, 12, 1647-1661.
- Adeyemi OS, Eseola OA, Plass W, Atolani O, Sugi T, Han Y, Batiha GE, Awakan OJ, Olaolu TD, Nwonuma CO, Alejolowo O, Owolabi A, Rotimi D, Kayode OT, Kato K. Imidazole derivatives as antiparasitic agents and use of molecular modelling to investigate the structure-activity relationship. Parasitol. Res., 2020, In press.
- Adeyemi OS, Sugi T, Han Y, Kato K. Screening of chemical compound libraries identified new anti-Toxoplasma gondii agents. Parasitol. Res., 2018a, 117(2), 355-363. doi: 10.1007/s00436-017-5698-1
- Adeyemi OS, Murata Y, Sugi T, Han Y, Kato K. Exploring amino acid-capped nanoparticles for selective anti-parasitic action and improved host biocompatibility. J. Biomed. Nanotechnol., 2018b, 14, 847-867.
- Adeyemi OS, Molefe NI, Awakan OJ, Nwonuma CO, Alejolowo O, Olaolu T, Maimako RF, Suganuma K, Han Y, Kato K. Metal nanoparticles show potential to restrict Trypanosoma growth. Artif. Cells, Nanomed.,
Biotechnol., 2018c, 46, S86-S94.
- Ishiwa A, Kobayashi K, Takemae H, Sugi T, Gong H, Recuenco FC, Murokoshi F, Inomata A, Horimoto T, Kato K. Effects of dextran sulfates on the acute infection and growth stages of Toxoplasma gondii. Parasitol. Res., 2013, 1-8.
- Adeyemi OS, Murata Y, Sugi T, Han Y, Kato K. Modulation of host HIF-1α activity and the tryptophan pathway contributes to the anti-Toxoplasma gondii potential of nanoparticles. Biochem. Biophys. Rep., 2017b, 11, 84-92.
- Emmanuel S. Solvent extraction and characterization of oil from African star apple (Chrysophyllum albidum) seeds. Acad. Res. Int., 2012, 3, 178-183.
- Aladekoyi G, Shakpo IO, Omosuli V, Jide AO. The effect of fermentation on the physico-chemical and the fatty acid composition of oil from raw and fermented African star apple endosperm (Chrysophyllum albidum). Int. J. Sci. Res. Eng. Stud., 2016, 3, 1-6.
- Kirk S, Sawyer R in Pearson’s Composition and Analysis of Foods, 9th Edition, Longman Gr. Ltd, Harlow, UK., 1991, p 708.
- Aremu MO, Ibrahim H, Bamidele TO. Physicochemical characteristics of the oils extracted from some Nigerian plant foods – A review. Chem. Process Eng. Res., 2015, 32, 36-52.
- Oyerinde AY, Bello EI. Use of Fourier transformation infrared (FTIR) spectroscopy for analysis of functional groups in peanut oil biodiesel and its blends. Br. J. Appl. Sci. Technol., 2016, 13, 1-14.
- Lautenschläger H. Essential fatty acids – Cosmetic from inside and outside. Beauty Forum, 2003, 2003, 54-56.
- Kanlayavattanakul M, Lourith N. Therapeutic agents and herbs in topical application for acne treatment. Int. J. Cosmet. Sci., 2011, 33, 289-297.
- Catalano A, Rodilossi S, Caprari P, Coppola V, Procopio A. 5-Lipoxygenase regulates senescence-like growth arrest by promoting ROS-dependent p53 activation. EMBO J., 2005, 24(1), 170-179.
- Dobrian AD, Ma Q, Lindsay JW, Leone KA, Ma K, Coben J, Galkina EV, Nadler JL. Dipeptidyl peptidase IV inhibitor sitagliptin reduces local inflammation in adipose tissue and in pancreatic islets of obese mice. Am. J. Physiol.: Endocrinol. Metab., 2011, 300(2), E410-21.
- Rackova L, Oblozinsky M, Kostalova D, Kettmann V, Bezakova L. Free radical scavenging activity and lipoxygenase inhibition of Mahonia aquifolium extract and isoquinoline alkaloids. J. Inflammation (London, U.K.), 2007, 4, 15.
- Eshwarappa RS, Ramachandra YL, Subaramaihha SR, Subbaiah SG, Austin RS, Dhananjaya BL. Anti-lipoxygenase activity of leaf gall extracts of Terminalia chebula (Gaertn.) Retz. (Combretaceae). Pharmacogn. Res., 2016, 8(1), 78-82.
- Fayemi SO, Osho A Atolani O. In vitro anticandidal and antioxidant potential of Mezoneuron benthamianum. J. Acute Dis., 2013, 1(2), 120-125. DOI: 10.1016/S2221-6189(13)60071-2
- Sokoudjou JB, Atolani O, Njateng GSS, Khan A, Tagousop CN, Bitombo AN, Kodjio N, Gatsing D. Isolation, characterization and in vitro antisalmonellal activity of compounds from stem bark extract of Canarium schweinfurthii. BMC Complementary Med. Ther., 2020, 20, 316.
- Sokoudjou JB, Tagousop CN, Khan A, Fodouop SPC, Atsafack SS, Kodjio N, Atolani O, Gatsing D. Canarimoic acid: New tirucallane triterpene with antisalmonellal activity from the stem bark of Canarium schweinfurthii Engl. Nat. Prod. Res., 2020, 36(9), 2363-2369. DOI:10.1080/14786419.2020.1836628
- Zubair MF, Atolani O, Ibrahim SO, Oguntoye OS, Abdulrahim HA, Oyegoke RA, Olatunji GA. Chemical and biological evaluations of potent antiseptic cosmetic products obtained from Momordica charantia seed oil. Sustain. Chem. Pharm., 2018, 9, 35-41.
- Zubair MF, Atolani O, Ibrahim SO, Oguntoye OS, Oyegoke RA, Olatunji GA. Fatty acids composition, antimicrobial potential and cosmeceutical utilization of Prosopis africana seed oil. J. Mex. Chem. Soc, 2018, 62(3).
- Dégbé M, Debierre-Grockiego F, Tété-Bénissan A, Débare H, Aklikokou K, Dimier-Poisson I, Gbeassor M. Extracts of Tectona grandis and Vernonia amygdalina have anti-Toxoplasma and pro-inflammatory properties in vitro (Les extraits de Tectona grandis et de Vernonia amygdalina ont des propriétés anti-Toxoplasma et pro-inflammatoires in vitro). Parasite (Paris), 2018, 25, 11.
- Gomes TC, Franco H, Júnior DA, Angélica S, Lescano Z. In vitro action of antiparasitic drugs, especially artesunate, against Toxoplasma gondii. Rev. Soc. Bras. Med. Trop., 2012, 45, 485-490.
- Choi W, Jiang M, Chu J. Antiparasitic effects of Zingiber officinale (Ginger) extract against Toxoplasma gondii. J. Appl. Biomed., 2013, 11, 15-26.
- Leesombun A, Boonmasawai S, Shimoda N, Nishikawa Y. Effects of extracts from Thai Piperaceae plants against infection with Toxoplasma gondii. PLoS ONE, 2016, 11(5), e0156116.
- Montazeri M, Mirzaee F, Daryani A, Naeimayi R, Moradi Karimabad S, Khalilzadeh Arjmandi H, Esmaealzadeh N, Shahani S. Anti-Toxoplasma activities of the hydroalcoholic extract of some Brassicaceae species. Adv. Biomed. Res., 2020, 9, 5.
|
|
|