|
|
|
The Chemist Volume 95 | Number 1 |

|
|
Starch and Coniferyl Alcohol Based Polymer: A Step Ahead Towards Green Polymers
|
|
Abstract: Enzymatic polymerization can be regarded as an environment friendly synthetic process of developing polymeric materials, providing a good example of green polymer chemistry. The present study depicts the polymer based on coniferyl alcohol and starch by using the enzyme in Pleurotus ostreatus mushroom powder, which acted as a versatile peroxidase to catalyze the reaction of polymerization. To observe the changes in bond formations, we take account of a comparison between IR spectra of starch and obtained samples of polymer. The IR characterization of the polymer synthesized shows some extra bands at 860 cm-1 and 709 cm-1, which shows the presence of some extra bond formation due to additives. The thermo-gravimetric curve shows continuous loss of mass with an increase in temperature. The DSC curve of polymer presented the glass transition temperature at approximately 80°C. The SEM analysis of starch film and the polymer shows the change in morphological pattern.
Key Words: Coniferyl alcohol, starch, biodegradability, polymer, thermo-gravimetric analysis
|
|
|
Introduction
Environmental concerns are rapidly growing over the large amounts of accumulating waste. Interest in biodegradable polymers has also risen sharply. Natural polymers can be sourced abundantly from renewable resources, while synthetic polymers originate from finite resources. Biodegradation of polymeric biomaterials involves cleavage of hydrolytically or enzymatically sensitive bonds in the polymer. Biodegradable polymers constitute a distinct category of polymers that undergo bacterial decomposition, yielding natural by-products like gases, water, biomass, and inorganic salts, and can be sourced from both natural and synthetic origins.
A vast study of literature shows that Vega et al (1996) performed the study of starch-based blends by thermo-gravimetric analysis and introduced a model that facilitates a precise understanding of material degradation exhibiting a symmetric curve in weight loss derivative concerning temperature. This model serves as a valuable instrument for analyzing blends in thermo-gravimetric studies, especially those comprising elements with significant temperature overlap during degradation [1]. Further study was carried by Onnerud et al. (2002) synthesized lignin oligomers. In a novel approach, peroxidase does not directly interact with lignin monomers such as coniferaldehyde and coniferyl alcohol. Instead, manganese oxalate functions as a diffusible redox shuttle. Initially, it is oxidized from manganese, Mn(II) to Mn(III), by a peroxidase, while simultaneously, the lignin monomers undergo oxidation to radicals, forming covalent linkages typical of lignin. Furthermore, a high molecular mass polymer is produced through the oxidation of coniferyl alcohol by Manganese(III) acetate in a mixture of dioxane and water [2]. In 2007, Barikani et al. investigated the synthesis and characterization of starch modified polyurethane and reported that the effect of prepolymer percentage on hydrophobicity was measured through contact angle and it was found that it increased with increasing amount of prepolymer. Glass transition temperature (Tg) is also affected with increasing amount of urethane linkage. By SEM, it was observed that the surfaces of modified starch are rougher and more disordered than the surface of unmodified starch particles, which confirms the grafting and modification of starch [3]. Kaewtatip et al. (2007) performed the preparation of cassava starch grafted with polystyrene by suspension polymerization. This study demonstrated the capability of polymerization of styrene monomer on the granular starch without emulsifier and the synthesis of graft copolymer without gelatinization of starch [4]. Su et al. (2012) presented the characterization of poly-caprolactone and starch blends for potential application within the biomaterials field. The findings revealed notable enhancements in the phases of PCL and starch with the application of MDI. Optimal results were achieved when the MDI content was at 1.0 wt% in conjunction with a starch content of 30 wt%. This proportion yielded satisfactory end products characterized by a smooth microstructure, appropriate mechanical properties, and high dynamic mechanical performance at body temperature scale [5]. Taboada-Puig et al. (2012) depicted the polymerization of coniferyl alcohol by a versatile peroxidase from Bjerkandera sp. and effects of H2O2 concentration aqueous organic solvents and pH. The aim of this study was to evaluate the ability of one versatile peroxidase and the bio-catalytically generated complex Mn(III) –malonate to polymerize coniferyl alcohol to obtain dehydrogenated polymers(DHPs) and to characterize the DHPs formed. Hydrogen peroxide was used as an oxidant and Mn2+ as a mediator. The results obtained from the Mn(III) –malonate-mediated polymerization showed that the yield was almost 100% [6]. In 2017, Ganguly et al. proposed the work on starch functionalized biodegradable semi-IPN as a pH tunable controlled release platform for memantine Thermal stability and degradation of the hydrogel was investigated through thermo-gravimetric analysis [7].
The vast study of starch and some different starch-based blends is also reported by multiple research workers. Starch is a highly hydrophilic macromolecule. It is often used as the degradable additive in the preparation of biodegradable polyethylene film. Polyethylene is resistant to microbial breakdown [8]. The challenge posed by polyethylene's non-degradability is a significant global issue. Consequently, scientists have explored various combinations involving starch and polyester/PVA/PCL compounds, which have been identified as biodegradable and mechanically compatible. PCL, a prominent biodegradable polyester widely used in biomedical applications, faces limitations in tissue regeneration due to its hydrophobic nature, potentially hindering cell adhesion and degradation rate. Blending PCL with starch, considered an optimal solution, can mitigate its hydrophobicity issue. Although naturally incompatible due to thermodynamic reasons, incorporating MDI as a compatibilizer in initial studies has shown improved interfacial interaction, thus enhancing the mechanical properties of PCL and starch blends [9].
Starch is a highly hydrophilic macromolecule which is used as the degradable additive in multiple blends. Starch and polyester blends are found to be biodegradable as both components are also biodegradable and compostable. When we use the starch filler with polyester, then it improves the rate of degradation of polymers [10,11]. In the study of degradation, scientists mentioned that enzymes like poly-(hydroxybutyrate) depolymerases and lipases are capable of cleaving the ester bonds [12,13]. As polyvinyl alcohol is hydrophilic in nature which makes it suitable to blend with starch. Investigation found that the PVA and starch blends have better mechanical properties [8].
From the vast body of literature of 20 years, it has been observed that coniferyl alcohol-based polymers were scarce. Therefore, the present article depicts the synthesis of starch and coniferyl alcohol-based polymer can prove to be a green polymer.
Experimental
Coniferyl alcohol (98%, Merck), starch (Emparta, Merck), MnSO4 (Emplura, Merck), mushroom powder (laboratory grade, Urban Platter), Hydrogen peroxide (laboratory grade, Merck), sodium malonate (for microbiology, Research Lab Fine Chem Industries), 1,4- dioxane (Emplura, Merck).
Coniferyl alcohol (98%, Merck), mushroom powder (laboratory grade, Urban Platter), and starch (Emparta, Merck) were used as received. 0.03 mole of coniferyl alcohol was dissolved in a 20% mixture of 1,4-dioxane and sodium malonate buffer (pH 6.5) to a total volume of 6 ml and placed in a syringe. Another syringe contained 6 ml of a mixture of H2O2 (33.3 mM) and MnSO4 (66.6 mM). Both solutions were added dropwise over 3 hours into a 250 ml conical flask containing 10 ml of an aqueous mushroom powder solution. Starch was dissolved in distilled water and heated to 80°C for 30 minutes to form a slurry, which was then added to the conical flask. The entire mixture was stirred using a magnetic stirrer for 24 hours. The resulting compound, which had a vanilla-like scent, was then oven-dried at 50°C for 24 hours, yielding a polymer film. This process synthesizes a polymer of coniferyl alcohol and starch using a mixture of 1,4-dioxane, sodium malonate, hydrogen peroxide, and manganese sulphate. The mushroom powder facilitates the enzymatic polymerization of coniferyl alcohol with starch. After 24 hours of stirring at room temperature, the mixture was centrifuged, and the resulting polymer was collected and oven dried.
Results and Discussion
The IR spectrum of formed polymer was recorded on a Vertex 70 (Bruker) instrument using a KBr pellet over a spectral range of 8,300—350 cm-1 and a maximum resolution of 0.5 cm-1 equipped with detector LaTiO3. Thermo-gravimetric analysis was carried on using the TGA V5/A Dupont 2100 at a heating range of 10°C/min under a nitrogen atmosphere in an alumina pan. Measurements of glass transition temperature were carried out with a differential scanning calorimeter general V2.2A Dupont 9900 model. DSC curves were recorded under a nitrogen atmosphere at a flow rate of 10°C/min. Scanning electron microscopy is an excellent tool for determining the morphology of the polymers. It was carried out in a CARL ZEISS EVO 50 TUNGSTEN SEM with the resolution of 2.0 nm at 30 kV and the magnification capacity was in between 5.00X and 10.00KX. Samples were mounted on a SEM coating unit for scanning.
In Figure 1, the narrow band was shown to be at 3507.15 cm-1, which shows the presence of group OH stretching.
Then, a band was obtained at 2141.27 cm-1 due to presence of group C-C triple bond stretching. At 1649.02 cm-1, a band was obtained which showed the presence of C=O stretching (Ϩ-lactum). At fingerprint region, in between 1500-667 cm-1, we obtain the bands of 1452.8 cm-1, 1370.87 cm-1, 1048.84 cm-1 and 924.8 cm-1, which are present due to C-H bending of alkane, C-H bending of aldehyde O=C-O-C=O anhydride stretching group and trans=C-H out of plane bending group.
In the IR analysis of the mushroom powder, we found a band at 3382 cm-1 which shows the presence of a H-bonded alcohol stretching group. Further, the obtained bands at 2926 cm-1, 2128 cm-1 and 1650 cm-1 depict the presence of aldehyde group having C-H stretching, C=C stretching in ring, and C=C stretching in alkenes, respectively. The bands situated at 1373 cm-1 and 1414 cm-1 show the presence of nitro stretching and sulphate stretching. We also found the bands at 1250 cm-1, 1203 cm-1, 1152 cm-1 and 574 cm-1 that show the presence of C-O stretching, tertiary saturated alcohol, C-O stretching and C-H bending in aromatic compounds, respectively. Some bands were also obtained at 1079 cm-1 and 1043 cm-1, which shows the presence of secondary alicyclic five or six membered ring. The IR analysis of the enzyme is shown in Figure 2.
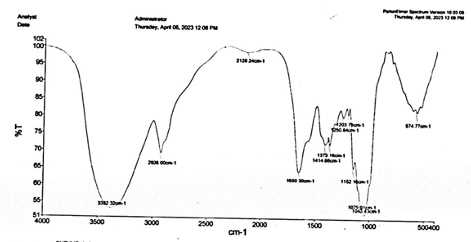 |
Observing the sample, we found signals in the 80% to 100% transmittance region. The first band was obtained at 3416.97 cm-1 which is also broader than the band of starch film. This band shows the presence of alcohol group OH stretching (intermolecular bonded). Another band was obtained at 2933.37 cm-1 which is completely invisible in the starch film, so it shows some combination in our polymer and shows the presence of the group C-H stretching of alkane. The band situated at 2154.48 cm-1 and this band are also broader than the starch film which is due to C=C=O stretching of ketene. A band was obtained at 1655.08 cm-1 which is narrower than the starch film band approximately near to this region which shows C=C stretching of vinylidene. In the finger print region, 1500-667 cm-1, the polymer sample shows the band at 1458.98 cm-1, 1364.53 cm-1, 1016.45 cm-1, 860.49 cm-1 and 709.93 cm-1, which shows the presence of C-H bending of methyl group, OH bending of phenol, OH bending of phenol, C-H bending of 1,4- di substituted or 1,2,3,4- tetra substituted alkane and benzene derivative. The bands obtained at 860.49 cm-1 and 709.93 cm-1 are absent in starch film, so these are new bands. The IR analysis of the polymer is shown in Figure 3.
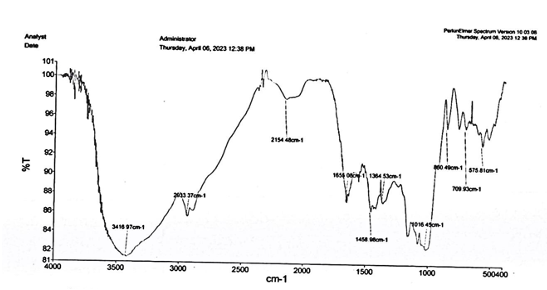 |
TGA analysis was carried on the V5/A Dupont 2100 at a heating range of 10°C/min under a nitrogen atmosphere in an alumina pan. The thermos-gravimetric results depict a loss in the mass on increasing the temperature. The polymer was stable up to 40°C and started losing weight above this temperature. We noted a continuous decomposition until 160°C (Figure 4).
On studying the curve obtained by the data, the phase change in the polymer sample started from 60°C and showed increasing heat flow from 100°C, so the glass transition temperature will occur at 80°C (Figure 5).
From the analysis of Figure 6, it can be seen that the surface of starch films without any additive was smooth, but as the content of additives were added to film, it changed its morphological pattern, which is shown in Figure 7.
Conclusion
Starch is an easily available, renewable and cheap material. It can be easily physically and chemically modified. Coniferyl alcohol is the precursor of lignin, which can be bonded to starch, and it can enhance the mechanical properties of polymer. The IR spectra of obtained film and starch film show the difference in their transmittance region, some new bands, and changes in the broadness of bands which represents the change in bonding patterns. In thermo-gravimetric analysis of the polymer, we found the continuous degradation of the polymer with the increase in temperature. DSC study of the graph shows the glass transition temperature at 80°C. SEM study showed the new morphological pattern found in the polymer film previously not reported in starch film.
References
- Vega D, Villar MA, Fallia MD, Valles EM. Thermo gravimetric analysis of starch based biodegradable blends. Polym. Bull.,1996, 37, 229-235.
- Onnerud H, Zhang L, Gellerstedt G, Henriksson G. Polymerization of mono-lignols by redox shuttle mediated enzymatic oxidation. Plant Cell, 2002, 14, 1953-1962.
- Barikani M, Mohammadi M. Synthesis and characterization of starch modified polyurethane. Carbohydr. Polym., 2007, 68, 773-780.
- Kaewtatip K, Tanrattnakul V. Preparation of cassava starch grafted with polystyrene by suspension polymerization. Carbohydr. Polym., 2007, 73, 647-655.
- Su J, Chen L, Li L. Characterization of poly-caprolactone and starch blends for potential application within the biomaterials field. Afr. J. Biotechnol., 2012, 11(3), 694-701.
- Taboada-Puig R, Lu-Chau TA, Tamminen T. Polymerization of coniferyl alcohol by a versatile peroxidase from Bjerkandera sp.: Effects of H2O2 concentration aqueous organic solvents and pH. Proceeding at the 4th International Conference on Pulping, Papermaking and Biotechnology, 2012, vol I&II. https://bit.ly/4e0DjVj
- Ganguly S, Maity T, Mondal S, Das P, Das NC. Starch functionalized biodegradable semi-IPN as a pH-tunable controlled release platform for memantine. Int. J. Biol. Macromol., 2017, 95, 185-198.
- Shujun W, Jiugao Y, Jinglin Y. Preparation and characterization of compatible and degradable thermoplastic starch/polyethylene film. J. Polym. Environ., 2006, 14, 1.
- Tang S, Zou P, Xiong H, Tang H. Effect of nano-SiO2 on the performance of starch/polyvinyl alcohol blend films. Carbohydr. Polym., 2008, 72, 521.
- Tokiwa Y, Suzuki T. Hydrolysis of polyesters by lipases. Nature, 1977, 270, 76.
- Ratto JA, Stenhouse PJ, Auerbach M, Mitchell J, Farrell R. Processing, performance and biodegradability of a thermoplastic aliphatic polyester/starch system. Polymer, 1999, 40, 6777.
- Kanesawa Y, Tanahashi N, Kumagai Y. Biodegradation of microbial polyesters in the marine environment. Polym. Degrad. Stab., 1992, 36, 173.
- Kumagai Y, Doi Y. Enzymatic degradation of poly(3-hydroxybutyrate)-based blends: Poly(3-hydroxybutyrate)/poly(ethylene oxide) blend. Polym. Degrad. Stab., 1992, 35, 87.
|