|
|
|
The Chemist Volume 92 | Number 1 |

|
|
|
Polyaniline Nanofibers: From Laboratory Curiosity to Commercial Products
|
|
Abstract: My research group began exploring nanostructured polyaniline nearly 20 years ago, right after I heard a lecture given by Dr. Bruce Weiller, an expert in sensors from Aerospace Corp. Bruce explained how the U.S. Air Force was interested in tracking the plumes left from rocket launches, especially as people moved closer to launch sites. One of the byproducts of burning solid rocket propellant is hydrochloric acid (HCl). Therefore, Bruce was interested in detecting HCl using a simple resistive-type sensor device. After Bruce’s seminar, I mentioned to him that we were working on the conjugated polymer, polyaniline, which on exposure to a strong acid would undergo over ten orders of magnitude increase in conductivity [1]. Bruce was intrigued and asked me to send him some polyaniline. About three weeks later, he called and mentioned he had both good and bad news. I said, “Please start with the good news,” to which he responded that the polyaniline my student had sent could readily detect HCl. Then I asked for the bad news. H e replied that the sensors were slow. Clearly what we needed was a high surface area form of our conjugated polymer.
Key Words: Polyaniline, PolyCeraÒ membranes
|
|
|
After discussing the problem with my research group, Jiaxing Huang (who is now a Professor of Materials Science and Engineering at Northwestern University) said that he would take care of this. Very quickly, Jiaxing developed a straightforward method to make nanostructured forms of polyaniline by using interfacial polymerization, an idea related to how Nylon is synthesized [2]. The ingredients for producing polyaniline are the aniline monomer combined with acid and oxidant, typically ammonium peroxydisulfate (APS). Mixing together all three ingredients produces a highly agglomerated form of polyaniline [3]. Jiaxing’s idea was to separate the aniline in an organic solvent from the acid and oxidant, which were dissolved in an aqueous solution. When one solution was placed on top of the other, the reaction kinetics were controlled by the interface, leading to nanofibers. In fact, Jiaxing demonstrated that when the acid was HCl, nanofibers formed with an average diameter of 30 nm and lengths on the order of microns [4,5,6]. Using camphor sulfonic acid produced polyaniline nanofibers with an average diameter of 50 nm, whereas perchloric acid created nanofibers with an average diameter of 120 nm, again with an average length on the order of microns. Note that polyaniline nanofibers had been previously produced by templated growth in zeolites [7] and anopore membranes [8]; however, removing these templates would be needed for applications such as sensors.
Testing by Bruce demonstrated that our new pure nanofibrillar form of polyaniline does indeed respond rapidly when exposed to acids. In fact, we observed a greater than six order of magnitude drop in resistance on exposure to 100 ppm of HCl when compared to the conventional agglomerated form of polyaniline [4]. We next developed ways of detecting weak acids such as hydrogen sulfide (H2S) by doping the polyaniline nanofibers with nanoparticles of copper chloride (CuCl2) [9]. Upon exposure to H2S, the dopants were converted to CuS and the strong acid HCl, which could readily be detected. By using more than one resistive sensor we could readily distinguish HCl produced from CuCl2 from HCl itself. In fact, in collaboration with the sensor start-up, Next Dimension, Inc., who used an array of 32 sensor heads, we were able to detect many deadly gaseous species in an effort supported by the U.S. Homeland Security Advanced Research Project Agency (HSARPA). Other deadly agents, such as hydrazine, phosgene and arsine, could be detected simply by adding other agents to the polyaniline nanofibers [10,11,12].
To detect bases such as ammonia, we used the HCl doped form of polyaniline [12,13]. Since these sensors were easy to make, we introduced them into the classroom, in collaboration with Prof. Sarah Tolbert, first for UCLA graduate students in Chem 285 and then for undergraduates in Chem 185. We even produced an instructor’s guide so that others can introduce conducting polymers and their use in sensors in their own classrooms [13].
Next, we studied the growth of metal nanoparticles on the polyaniline nanofibers. Since polyaniline is a redox active polymer, upon exposure to chloroauric acid (HAuCl4), the gold in its +3 oxidation state is reduced to gold zero. Since the gold nucleates uniformly across the nanofibers, the size of the gold nanoparticles can be controlled simply by varying the time, temperature and concentration of HAuCl4. By turning off the electrical conductivity with base, these nanostructured gold particles can be used in crossbar type memory devices as we demonstrated in collaboration with Prof. Yang Yang [14]. By applying a potential of >3 V, these devices exhibit a high conductivity on-state that is reversed upon application of -5 V to a low conductivity off-state. The mechanism appears to involve a reversible charge transfer from the gold to the polyaniline [15].
Prof. Paula Diaconescu, who is an expert in catalysis, then asked us to provide her with polyaniline nanofibers decorated with palladium nanoparticles. Her group then examined Suzuki coupling reactions in which aryl halides were shown to react with boronic acid in water with high yields at relatively low temperatures [16]. A semi-heterogeneous mechanism appears to be operative [17].
We then looked at what happens when polyaniline nanofibers are exposed to an intense beam of light. The answer is they melt in a process we termed flash welding [18]. By applying masks, we created interdigited electrodes that could be used for sensors and other electronic devices [19]. Working with Prof. Geoff Spinks and Prof. Gordon Wallace at the Intelligent Polymer Research Institute at the University of Wollongong while carrying out a Fulbright Fellowship in Australia, we controlled the penetration depth of the light to create asymmetric membranes that acted as mechanical actuators upon exposure to acids. Basically, the un-welded side expands when contacted by acid, while the welded side cannot, producing a rotation of up to 720 degrees within 20 seconds in an ∼10 cm long piece of flash welded nanofibrillar polyaniline [20]. The rotation is completely reversed on exposure to base.
I presented many of our discoveries on nanostructured polyaniline to UCLA professors at one of our inaugural meetings of the California NanoSystems Institute (CNSI). (Reviews of our work can be found in References 21-23.) After my lecture, Dr. Eric Hoek, then an Assistant Professor of Civil and Environmental Engineering, asked me if I had considered using nanostructured polyaniline for membrane separations. When I mentioned our seminal work on gas separations using traditional polyaniline [24], he was intrigued and wanted to test liquid separations. We began an extremely productive collaboration that has resulted in many advances in conjugated polymer-based membranes [25-32]. Our first major funding for this project came from Abraxis Biosciences headed by Dr. Patrick Soon-Shiong, when we proposed creating a membrane to speed up kidney dialysis. Within two years Eric and I had created a membrane that could accomplish comparable separations to kidney dialysis five times faster. When we asked for further funding to build actual devices, we were told that Patrick was only interested in companies, so we would need to start a company, put together a business plan and present it to his team (as Patrick was by then too busy to meet with us directly). Eric said we should do this as we both had experience with start-up companies. Eric’s first company NanoH2O, Inc. used nanoparticle additives to enhance reverse osmosis membranes [33-34]. NanoH2O was acquired in 2014 by the Korean company LG Chem for $200M. My first company Fibron, Inc. worked to scale the production of polyaniline nanofibers. We spent over four years perfecting the synthesis of polyaniline nanofibers using a rapid mixing technique as can be seen in Figure 1 [5,35].
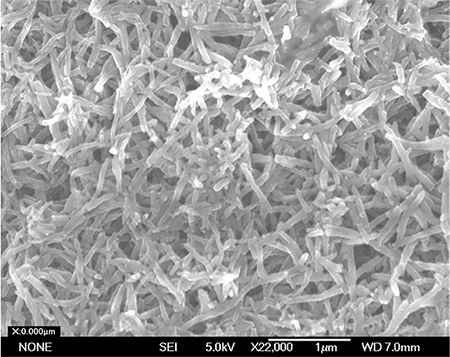 |
With help from the Korean company, Kolon, we demonstrated the ability to make polyaniline nanofibers on a pilot plant scale of 100 liters per batch. Unfortunately, during the European debt crisis in 2014 one of our European partners pulled out at the last minute of a $5.5 million funding deal with a venture capital group. When none of our initial investors offered to fill in the missing investment, the venture group reneged on the deal and we were forced to liquidate the company. Fortunately, the ‘use it or lose it’ clause for our intellectual property licensed from UCLA meant that the patents returned to UCLA. Several of these patents formed the basis for the new company Eric and I started called Polycera, Inc. Our name came from a combination of an inexpensive polymer membrane that acts like an expensive ceramic membrane. Unfortunately, when developing our business plan, we learned from a physician that the human body can’t take kidney dialysis at a faster speed then it is currently done. Although I was dumbfounded that we had not even considered this possibility when starting our project two years earlier, Eric had little hesitation in suggesting that there were even more important applications for our new membranes, such as cleaning up the mess created by the hydraulic fracturing used to produce oil, known as fracking. It turns out that for every barrel of oil obtained via fracking, 7-10 barrels of oily wastewater are produced; Eric suggested that our membranes could separate this oil from water. We presented our business plan and slide deck to Patrick’s associates and received a very positive response with the sentiment that with their blessing there was a 90% Patrick would fund our endeavor. While we waited for a final decision, Eric suggested we travel to Silicon Valley and present our ideas to real venture capitalists. This was an eye-opening experience – heading to Sand Hill Road in the heart of Silicon Valley and acting as bone fide entrepreneurs. Patrick then had an epitome in which he decided his funds should be used to further healthcare and while kidney dialysis fell nicely into this arena, clean water did not. In the meantime, we received an enticing offer for funding from a venture group in Silicon Valley. Eric started negotiations and then realized that another company he was involved with – Water Planet, LLC – could make use of our new membranes. Basically, Water Planet had developed a method to separate 99% of oil from water but needed a membrane to clean up the last 1%. They were currently using expensive ceramic membranes and realized that our membranes could handle the same problem much more efficiently. Water Planet offered to buy Polycera, Inc. for the price of the term sheet offered by the venture capital group. This was an offer that made a great deal of sense. So, while my first company Fibron, Inc. failed due to bad timing, my second start-up Polycera, Inc. succeeded due to good timing.
Water Planet went on to scale up our membranes and demonstrate that they reduced the costs associated with cleaning up the mess left from oil fracking by up to 40%. Water Planet liked our name and called their product PolyCeraÆ membranes. Within the past couple years, PolyCeraÆ membranes have been installed in over 100 oil installations around the world. Water Planet recently spun off PolyCera, Inc. as a separate company that is exploring the use of these membranes for other separations from fruit juice to milk products and beyond.
It has been quite a journey from the development of polyaniline nanofibers to solve a problem involving slow sensors to finding a method to clean up the mess created by oil fracking. I hope this article will provide inspiration for other aspiring entrepreneurs.
Acknowledgments
I very much want to thank my students including Jiaxing Huang and collaborators including Bruce Weiller, Yang Yang, Paula Diaconescu, Sarah Tolbert, Geoff Spinks, Gordon Wallace and especially Eric Hoek without whom none of this would have been possible. I also want to thank our sponsors, including the National Science Foundation, the Microelectronics Advanced Research Corp., Homeland Security Advanced Research Projects Agency, Abraxis Bioscience, Boeing and Water Planet.
References
- Chiang J-C, MacDiarmid AG. Synth. Met., 1986, 13, 193-205.
- DuPont, Nylon: A DuPont Invention, DuPont International, Public Affairs, 1988, pp 2–3.
- Huang J, Kaner RB. Chem. Commun., 2006, 367-376.
- Huang J, Virji S, Weiller BH, Kaner RB. J. Am. Chem. Soc., 2003, 125, 314-315.
- Huang J, Kaner RB. J. Am. Chem. Soc., 2004, 126, 851-855.
- Huang J, Kaner RB. Angew. Chem., Int. Ed., 2004, 43, 5817-5821.
- Wu C, Bein T. Science, 1994, 264, 1757-1759.
- Martin CR. Acc.Chem. Res., 1995, 28, 61-68.
- Virji S, Fowler JD, Baker CO, Huang J, Kaner RB, Weiller BH. Small, 2005, 1, 624-627.
- Virji S, Kaner RB, Weiller BH. Chem. Mater., 2005, 17, 1256-1260.
- Virji S, Kojima R, Fowler J, Kaner RB, Weiller BH. Chem. Mater., 2009, 21, 3056-3061.
- Virji S, Kojima R, Fowler JD, Villanueva JG, Kaner RB, Weiller BH. Nano Res., 2009, 2, 135-142.
- Virji S, Weiller BH, Huang J, Blair R, Shepherd H, Faltens T, Haussmann PC, Kaner RB, Tolbert SH. J. Chem. Ed., 2008, 85, 1102-1104.
http://www.jce.divched.org/Journal/Issues/2008/Aug/abs1102.html
- Tseng RJ, Huang J, Ouyang J, Kaner RB, Yang Y. Nano Letters, 2005, 1077-1080.
- Tseng RJ, Baker CO, Shedd B, Huang J, Ouyang J, Kaner RB, Yang Y. Appl. Phys. Lett., 2007, 90, 053101.
- Gallon BJ, Kojima RW, Kaner RB, Diaconescu PL. Angew. Chem., Int. Ed., 2007, 46, 7251-7254.
- Lemke WM, Kaner RB, Diaconescu PL. Inorg. Chem. Front. 2015, 2(35), 1-6.
- Huang J, Kaner RB. Nature Materials, 2004, 3, 783-787.
- Strong V, Wang Y, Patatanyan A, Whitten PG, Spinks GM, Wallace GG, Kaner RB. Nano Letters, 2011, 11, 3128-3135.
- Baker CO, Shedd B, Innis PC, Whitten PG, Spinks GM, Wallace GG, Kaner RB. Adv. Mater., 2008, 20, 155-158.
- Li D, Huang J, Kaner RB. Acc. Chem. Res., 2009, 42, 135-145.
- Tran HD, D’Arcy JM, Wang Y, Beltramo PJ, Strong V, Kaner RB. J. Mater. Chem., 2011, 21, 3534-3540.
- Baker CO, Huang X, Nelson W, Kaner RB. Chem. Soc. Rev., 2017, 46, 1510-1525.
- Anderson MR, Mattes BR, Reiss H, Kaner RB. Science, 1991, 252, 1412-1415.
- Guillen GR, Farrell TP, Kaner RB, Hoek EMV. J. Mater. Chem., 2010, 20, 4621-4628.
- Liao Y, Zhang C, Zhang Y, Strong V, Tang J, Li X-G, Kalanter-zadeh K, Hoek EMV, Kaner RB. Nano Lett., 2011, 11, 954-959.
- McVerry BT, Temple JAT, Huang X, Marsh KL, Hoek EMV, Kaner RB. Chem. Mater., 2013, 25, 3597.
- Liao Y, Wang X, Chian W, Yu D-G, Li X-G, Hoek EMV, Kaner RB. Nanoscale, 2013, 5, 3856-3862.
- Liao Y, Li X-G, Hoek EMV, Kaner RB. J. Mater. Chem. A, 2013, 1, 15390-15396.
- Liao Y, Farrell TP, Guillen GR, Li M, Temple JAT, Li X-G, Hoek EMV, Kaner RB. Mater. Horiz., 2014, 1, 58-64.
- Huang X, McVerry BT, Marambio-Jones C, Wong MCY, Hoek E, Kaner RB. J. Mater. Chem. A, 2015, 3, 8725-8733.
- McVerry BT, Anderson M, He N, Kweon H, Ji C, Xue S, Rao E, Lee C, Kaner RB. Nano Lett., 2019, 19(8), 5036-5043.
- Jeong BH, Hoek EMV, Yan Y, Huang X, Subramani A, Hurwitz G, Ghosh AK, Jawor A. J. Membr. Sci., 2007, 294, 1-7.
- Lind ML, Ghosh AK, Jeong BH, Hoek EMV. Langmuir, 2009, 25, 10139-10145.
- Li D, Kaner RB. J. Mater. Chem., 2007, 17, 2279-22782.
|