|
|
|
The Chemist Volume 92 | Number 1 |

|
|
Imidazo Pyridine Derivative as a New Corrosion Inhibitor for Mild Steel in Acidic Media
|
|
Abstract: The corrosion inhibition of mild steel (MS) in 0.5 M HCl and 1 M HCl solution by 2-(4-methylphenyl)imidazo[1,2]pyridine was studied at temperature range from 303-333 K by weight loss measurements. The results show that the studied compound exhibits good performance as an inhibitor for MS in both 0.5 and 1 M HCl solution. The inhibitor efficiency of the inhibitor was dependent on concentration as well as temperature of the solution. The adsorption on mild steel surface was explained through Langmuir’s adsorption isotherm model. The values of standard free energy of adsorption indicated adsorption of aminopyridine derivative was a spontaneous process and adsorbed chemically as well as physically on the metal surface.
Key Words: Mild steel, imidazo pyridine inhibitors, weight loss method, kinetic parameters, Langmuir adsorption
Ethics Approval
Not applicable as no involvement of human participants or any other biological objects.
Conflicts of Interest
The authors declare no conflict of interest.
|
|
|
Introduction
Mild steel is a key construction component in the petroleum industry and power plants. In most of the industrial processes, mineral acids are widely used for cleaning, pickling and descaling processes. In mineral acids, metallic corrosion represents a lamentable waste of both resources and money; they must be protected. As the dissolution of these components in an acid medium is very high, it may be desirable to use inhibitors to reduce the corrosive attack on metals [1].
Heterocyclic compounds having nitrogen, sulphur or oxygen atoms are of special significance as they often provide superior inhibition along with the presence of polar groups and/or π electrons [2]. These kinds of organic molecules are adsorbed on the surface of the metal, consequently controlling the rate of corrosion.
Imidazo pyridines are essential organic molecules used in the pharmaceutical industry for their antiviral [3], anti-inflammatory [4], anti-bacterial [5], analgesic, antipyretic and anticonvulsant [6] properties. The presence of a N-heterocyclic system makes them well-qualified, excellent inhibitors for mild steel corrosion in an acidic medium [7,8].
The present study explores the use of one of the imidazo pyridine derivatives namely 2-(4-methylphenyl)imidazo[1,2]pyridine as a corrosion inhibitor for mild steel surface in 0.5 and 1 M hydrochloric acid solution using gravimetric analysis. Owing to the simple application, accuracy and reliability, this method was chosen to understand the corrosion inhibitive effect of the inhibitor. The effect of solution temperature, concentration of the acid solution, as well as inhibitor concentration on the electrochemical dissolution of mild steel, were studied and discussed. Kinetic and thermodynamic parameters were also calculated and discussed.
Experimental Procedure
Test coupons: The weight loss study was performed on mild steel containing (in wt %) C- 0.18, Mn - 0.6, S - 0.05, P - 0.04, Si - 0.1 and Fe - 99.03. The mild steel coupons were mechanically cut, having dimensions 2 cm x 2 cm x 0.5 cm and polished with different grade of emery papers (220, 330, 500, 600, 800, 1000, 1200 grade) for the study. Before immersing in the test solution for the gravimetric study, mild steel coupons were washed with double distilled water, degreased in acetone and dried.
Test solutions: For the gravimetric study, the 0.5 and 1 M HCl electrolyte solutions were prepared by using analytical grade 37% HCl (Merk) solution and double distilled water.
Corrosion inhibitor: 2-(4-methylphenyl)imidazo[1,2-a]pyridine was used as a corrosion inhibitor for the present investigation. The concentration of the inhibitor used for investigation ranged from 50 - 200 ppm. The molecular structure of 2-(4-methylphenyl)imidazo[1,2-a]pyridine is shown in Figure 1.
Weight Loss Study: Weight loss measurement was carried out by immersion of precisely weighed mild steel test coupons in the absence and presence of 50, 100, 150 and 200 ppm of the inhibitor in 100 ml of 0.5 and 1 M HCl solution for 2 h. The test coupons were then retrieved from electrolyte, washed with distilled water followed by acetone, dried and reweighed using an analytical balance with the accuracy of ±0.1 mg. The tests were performed in a cell equipped with thermostatic cooling condenser at different temperatures (303 K, 313 K, 323 K and 333 K).
The rate of corrosion (CR), and inhibition efficiency (η%) are calculated using following equations 1 and 2 [9]:
where ∆w = weight loss in g, A = area of specimen in cm2 exposed in acidic medium, t = immersion time in h, and d = density of mild steel (g cm-3).
where CRo and CRi are corrosion rates for uninhibited and inhibited solution.
Result and Discussion
Corrosion inhibition efficiency (η%) of imidazo pyridine derivative and corrosion rate (CR) values calculated by weight loss measurements after 2 h of exposure to both the electrolyte solutions at varying temperatures (303-333 K) are listed in Table 1. The corrosion rates obtained were plotted against different inhibitor concentrations in 0.5 and 1 M HCl solutions are shown in Figures 2(a) and 2(b), respectively.
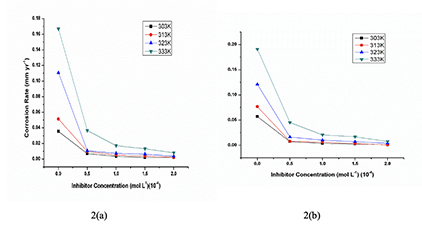 |
The data in Table 1 shows that rate of corrosion was much less in the inhibited solution than that of the uninhibited solution. These experimental values prove that as concentration of acid increases, the corrosion inducing property also increases, thereby increasing the corrosion rate of the MS. However, the corrosion rate decreases in the presence of inhibitor, clearly indicating the inhibitive action of imidazo pyridine derivative on corrosion behaviour of mild steel in acidic medium. The inhibition effect could be attributed to the adsorption of the inhibitor molecules/ions from the electrolyte solution at the metal surface. The greater inhibition effect at higher concentration of the inhibitor may be due to an increased rate of adsorption rather than desorption/dissolution of the imidazo pyridine derivative at the metal surface that leads to larger surface area coverage. Thus, the corrosion product formed acts as a protective film that increases the charge transfer resistance at the solid/electrolyte interface region [10].
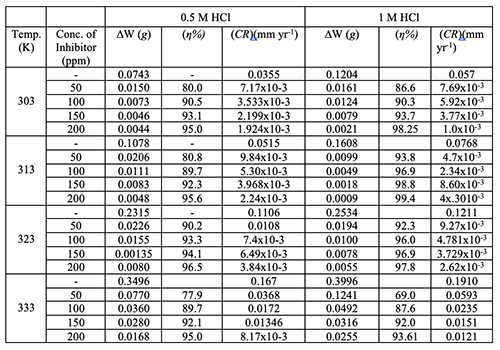 |
Analysis of inhibition efficiencies at various concentrations of inhibitor at the studied range of temperatures can give us some useful insight into the possible inhibition mechanism of the inhibitor. Table 1 shows variation of corrosion rate with temperature (303-333 K) in 0.5 and 1 M HCl solution in the absence and presence of 2-(4-methylphenyl)imidazo[1,2-a]pyridine.
The data indicate that corrosion rate of the MS increases with increasing temperature in the absence of inhibitor. But, in the presence of inhibitor, inhibition efficiency (η%) increases by different amounts at different concentrations of the inhibitor at constant temperature and correspondingly the corrosion rate decreases. But an interesting trend of inhibition was observed when temperature of the medium was increased. In 0.5 M HCl solution, η% increased with increasing inhibitor concentration from 303 K to 323 K; however, further raising the temperature did not show noticeable improvement in inhibition performance of the inhibitor. Whereas, in 1 M HCl the enhanced inhibition performance of the inhibitor was seen with the increasing temperature up to 313 K, thereafter inhibitive effect decreased. Hence, based on the above experimental evidence it can be ascertained that the tested imidazo pyridine derivative may serve as a potential inhibitor to protect mild steel metal from acidic attack.
Table 1 shows the overall inhibition efficiency is high for 1 M HCl compare to 0.5 M HCl. This may be due to the ability of the inhibitor to form a protonated complex in an acidic medium, which mainly depends on nature and concentration of the electrolyte and inhibitors. The inhibitor imidazo pyridine derivative in acidic media protonates to form a protonated complex and its population is governed by acid and inhibitor concentration. Compared to 0.5 M HCl, in 1 M HCl solution, the number of protonated species of inhibitor imidazo pyridine derivative is high; Cl- species are also increased. The Cl- ions are not only adsorbed by the positively charged iron surface but also facilitate the adsorption process of the protonated inhibitor through an intermediate bridge between the metal and end of protonated inhibitor. Thus, protonated inhibitor species along with the Cl- ions block the electrochemical active sites of the metal surface and hinder the metal dissolution rate [11].
Generally, with the effect of temperature, the corrosion of metal in acid media is accompanied by the evolution of hydrogen gas, which is followed by an increase in the metal dissolution process. The effect of temperature on the inhibited metal is a very complex reaction due to rapid desorption reaction of inhibitor on metal surface or decomposition of inhibitor itself [12]. The mechanism of inhibition can be deduced by comparing the Arrhenius equation in the presence and absence of inhibitor. This relationship can be expressed in terms of Arrhenius equation (3)
[13-16], where CR is the corrosion rate, Ea is apparent activation energy, R is the molar gas constant (8.314 J mol-1 K-1), T is absolute temperature and A is Arrhenius factor.
A plot of log CR versus 1/T gave a straight line for mild steel in 0.5 M HCl and 1 M HCl solution in the presence and absence of different concentrations of inhibitor as shown in Figure 3(a) and 3(b).
The activation energy values (Ea), calculated from the slope of the straight line (-Ea/2.303R) for 0.5 and 1 M HCl solutions, are given in Table 2.
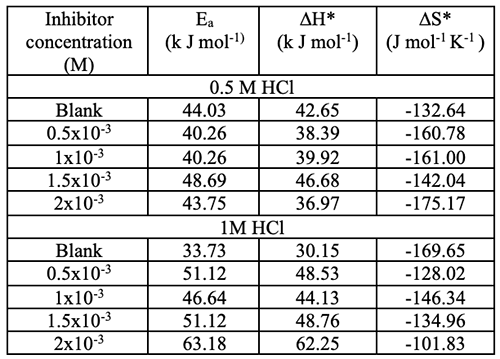 |
Generally, lower values of Ea in the inhibited system compared to the blank indicate a chemisorption mechanism, whereas higher values of Ea suggest physisorption [17]. The data in Table 2 shows that in 0.5 M HCl solution, Ea values are less in the presence of lower concentrations of inhibitor compared to uninhibited solution, whereas at higher concentrations these values are slightly more or equal to that of the blank solution. As a result of this, it could be attributed to chemical adsorption at lower concentrations of the inhibitor due to the formation of a strong co-ordination bond between d orbital of iron and inhibitor molecule [18], which controls the charge transfer on the mild steel metal surface. At higher concentrations, physisorption is more likely to take place. But in the case of 1 M HCl, the calculated Ea value for uninhibited solution is less compared to inhibited solution and the range of Ea values (33.73 kJ mol-1 to 63.18 kJ mol-1) are lower than the threshold value of 80 kJ mol-1 which is required for chemisorption, indicating physisorption takes place [19].
In general, the physisorption process requires the presence of both an electrically charged metal surface and charged inhibitor species in the solution. In the case of chemisorption, this occurs either by charge transfer or by a sharing pair of electrons between a metal atom and inhibitor species [11]. In the present study, the inhibitor imidazo pyridine, which is an organic molecule, protonates in the presence of acidic media, forming cations which exist in equilibrium with corresponding molecular forms, resulting in physical adsorption. This can be explained by considering the continuous process of adsorption and desorption of inhibitor molecules at the metal surface, which is an equilibrium process at a particular temperature. With the increase of temperature, an equilibrium shift takes place leading to a higher desorption rate than adsorption until equilibrium is regained [20]. This explains the decrease in inhibition efficiency with increase in temperature (physical adsorption), i.e., weak Vander Waal’s forces, which disappears at elevated temperatures [21,22]. Thus, in both 0.5 and 1 M HCl solutions inhibition efficiencies increased up to a certain level of temperature, afterwards decreasing, showing a lower reactivity of the inhibitor may be due to desorption or dissolution of the substrate from the metal surface. The enthalpy and entropy of activation for the metal corrosion process are determined using the transition state equation (4) [12].
 |
where h is Plank’s constant (6.626176 x 10-34 J s, N is Avogadro’s number (6.02252 x 1023 mol-1), R is the universal gas constant and T is the absolute temperature.
Figure 4(a) and 4(b) show the plot of log CR/T versus 1/T for corrosion of mild steel in 0.5 M HCl and 1 M HCl solution in the absence and presence of various concentrations of imidazo pyridine derivative, respectively.
Straight lines with slope of and an intercept of were obtained from which the values of ∆H*1 and ∆S* were calculated and listed in Table 2 for corrosion of mild steel in 0.5 and 1 M HCl solutions. This investigation shows that the positive value of ∆H* indicates that corrosion of mild steel in HCl solution is an endothermic process [23]. Large and negative values of entropies (∆S*) for both inhibited and uninhibited solution imply that the rate determining step, activation complex, is with association rather than dissociation [24], which results in a decrease of randomness on going from reactants to the activated complex.
The electrochemical reaction that takes place on the metal surface can be related to adsorption of the inhibitor, which depends on the nature of the metal surface, electronic characteristics of the metal surface on adsorption, temperature, and electrochemical potential at the metal solution interface [25]. It is a surface phenomenon and can be determined by experimental data.
Electron donor atoms like N, S or O in the molecular structure of the inhibitor favours the greater adsorption on the metal surface. Thus, the adsorption isotherm gives information on the metal-inhibitor interactions. To get the isotherm, the linear relation between degree of surface coverage (θ) values (θ= η% /100) and inhibitor concentration Cinh must be determined since surface coverage values are very useful in measuring the adsorption characteristics.
Several attempts were made for various isotherms like Langmuir, Temkin, Frumkin, Flory-Huggins, etc. to fit the θ values. In the present study, the experimental data were best fitted by the Langmuir adsorption isotherm. According to this isotherm, θ is related to Cinh using the following equations (5 and 6).
Rearranging equation 5 gives:
where Kads is the equilibrium constant of the inhibitor in adsorption/desorption process,Cinh is the concentration of the inhibitor and θ is the surface coverage area. The graph of Cinh / θ versusCinhwas plotted and fitted straight line was obtained with a slope close to unit as seen in Figure 5a and 5b.
The correlation factor R2> 0.9999 indicating that adsorption of inhibitor on mild steel follows the Langmuir adsorption isotherm. According to this isotherm, adsorbed molecules occupy only one site and there are no interactions with the other adsorbed species [26,27]. The calculated parameters such as equilibrium constant Kads linear regression coefficient and slopes for 0.5 and 1 M HCl solutions are listed in Table 3.
A larger value of Kads shows the more efficient adsorption, hence the better inhibition efficiency [28]. It is evident from the data in Table 5 that, Kads value decreases with increase of temperature showing adsorption of inhibitor 2-(4-methylphenyl)imidazo[1,2]pyridine on mild steel is unfavourable at higher temperature.
The equilibrium constant Kads is related to the standard free energy of adsorption (ΔG0ads ) using the following equations (7 and 8):
where R is the gas constant and T is the absolute temperature and 55.5 is the molar concentration of water in solution [29]. The (ΔG0ads ) values calculated for the inhibitor in 0.5 and 1 M HCl solutions are summarized in Table 5. The negative values for free energy of adsorption (ΔG0ads ) show the spontaneity of adsorption process [30] and also stability of adsorbed layer on the mild steel surface.
Generally, the value of (ΔG0ads ) up to -20 kJ mol-1 is consistent with physisorption, while those around -40 kJ mol-1 or higher associated with chemisorption as a result of sharing or transfer of electrons from inhibitor organic molecule to the metal surface to form a strong coordination bond [30].
In the present case, values for 2-(4-methylphenyl)imidazo[1,2a]pyridine inhibitor on mild steel in presence of 0.5 M HCl and 1 M HCl solution ranges from -38.29 kJ mol-1 to -42.33 kJ mol-1 and -38.86 kJ mol-1 to -42.81 kJ mol-1, respectively. This reveals that the process of adsorption involves both physisorption and chemisorptions in particular, the former dominating over the latter. The absolute value of (ΔG0ads ) increases with an increase in temperature, indicating that adsorption becomes more favourable and also that inhibitor molecules are chemically adsorbing on the mild steel metal surface.
Thermodynamic parameters are important in studying adsorption of organic inhibitor on a metal surface. The enthalpy of adsorption (ΔH0ads ) and entropy of adsorption (ΔS0ads ) are deduced by a thermodynamic equation (9):
The standard enthalpy of adsorption (ΔH0ads ) and standard entropy of adsorption (ΔS0ads ) are -3.329 kJ mol-1, 0.1379 J mol-1 K-1, in 0.5 M HCl and -6.225 kJ mol-1, 0.1835 J mol-1 K-1, respectively. The negative values of (ΔS0ads ) indicate that decrease in randomness takes place on going from reactant to metal-adsorbed species.
From the results, it can be concluded that the tested amine derivative, namely 4-methyl imidazo pyridine, shows encouraging inhibitive action on corrosion of mild steel in acidic media. The electrochemical reactive sites formed on the metal surface on exposure to aggressive electrolyte were believed to be effectively blocked by the inhibitor molecules through the adsorption mechanism. The calculated (ΔG0ads ) values for the inhibitor in both 0.5 and 1 M HCl solutions indicate mixed adsorption with predominant involvement of physisorption. The adsorption was spontaneous and exothermic and best described by the Langmuir adsorption model. The variation of corrosion rates and inhibition efficiencies with the concentration of acid, inhibitor and solution temperatures shows the inhibition tendency of the studied amine derivative on the mild steel surface was controlled by concentration and temperature of the solution.
- Bentiss F, Traisnel M, Lagrenee M. Corros. Sci., 2000, 42(1), 127–146.
- Mernari B, El Attari H, Traisnel M, Bentiss F, Lagrenee M. Corros. Sci., 1998, 40(2–3), 391–399.
- Gueiffier A, Mavel S, Lhassani M, Elhakmaoui A, Snoeck R, Andrei G, Chavignon O, Teulade J-C, Witvrouw M, Balzarini J, De Clercq E, Chapat J-P. J. Med. Chem., 1998, 41(25), 5108–5112.
- Rupert KC, Henry JR, Dodd JH, Wadsworth SA, Cavender DE, Olini GC, Fahmy B, Siekierka JJ. Bioorg. Med. Chem. Lett., 2003, 13(3), 347–350.
- Rival Y, Grassy G, Michel G. Chem. Pharm. Bull., 1992, 40(5), 1170–1176.
- Almirante L, Polo L, Mugnaini A, Provinciali E, Rugarli P, Biancotti A, Gamba A, Murmann W. J. Med. Chem., 1965, 8(3), 305–312.
- Vranda KS, Kumari PDR. 2020, p 040006.
- Salim R, Ech-chihbi E, Oudda H, El Hajjaji F, Taleb M, Jodeh S. J. Bio. Tribo. Corros., 2019, 5(1), 14.
- Ji G, Shukla SK, Dwivedi P, Sundaram S, Prakash R. Ind. Eng. Chem. Res., 2011, 50(21), 11954–11959.
- Ravichandran R, Nanjundan S, Rajendran N. J. Appl. Electrochem., 2004, 34(11), 1171–1176.
- Popova A, Sokolova E, Raicheva S, Christov M. Corros. Sci., 2003, 45(1), 33–58.
- Bentiss F, Lebrini M, Lagrenée M. Corros. Sci., 2005, 47(12), 2915–2931.
- Khedr MGA, Lashien AMS. Corros. Sci., 1992, 33(1), 137–151.
- Schorr M, Yahalom J. Corros. Sci., 1972, 12(11), 867–868.
- Umoren SA, Solomon MM, Udosoro II, Udoh AP. Cellul., 2010, 17(3), 635–648.
- Solomon MM, Umoren SA, Udosoro II, Udoh AP. Corros. Sci., 2010, 52(4), 1317–1325.
- Morad MS, El-Dean AMK. Corros. Sci., 2006, 48(11), 3398–3412.
- Chaieb E, Bouyanzer A, Hammouti B, Benkaddour M. Appl. Surf. Sci., 2005, 246(1–3), 199–206.
- Hamdy A, El-Gendy N Sh. Egypt. J. Pet., 2013, 22(1), 17–25.
- Jamal Abdul Nasser A, Anwar Sathiq M. Arabian J. Chem., 2016, 9, S691–S698.
- Obi-Egbedi NO, Obot IB. Arabian J. Chem., 2012, 5(1), 121–133.
- Obot IB, Obi-Egbedi NO, Umoren SA. Corros. Sci., 2009, 51(2), 276–282.
- Mu, GN, Li X, Li F. Mater. Chem. Phys., 2004, 86(1), 59–68.
- Soltani N, Behpour M, Ghoreishi SM, Naeimi H. Corros. Sci., 2010, 52(4), 1351–1361.
- Villamil RFV, Corio P, Agostinho SML, Rubim JC. J. Electroanal. Chem., 1999, 472(2), 112–119.
- Dehri İ, Özcan M. Mater. Chem. Phys., 2006, 98(2–3), 316–323.
- Eddy NO, Odoemelam SA, Odiongenyi AO. J. Appl. Electrochem., 2009, 39(6), 849–857.
- Lagrenée M, Mernari B, Bouanis M, Traisnel M, Bentiss F. Corros. Sci., 2002, 44(3), 573–588.
- Quraishi MA, Ahamad I, Singh AK, Shukla SK, Lal B, Singh V. Mater. Chem. Phys., 2008, 112(3), 1035–1039.
- Tang L, Li X, Li L, Mu G, Liu G. Mater. Chem. Phys., 2006, 97(2–3), 301–307.
|
|