|
|
|
The Chemist Volume 94 | Number 2 |

|
|
Eco-Friendly Formulation, Characterizations, Bioactivity Studies and in silico Evaluation of Cosmetic prepared from the Seed Oils of Carica papaya, Dacryodes edulis and Raphia hookeri
|
|
Abstract: A quarter of a century ago, there was a renewed interest in the application of natural products in cosmetic formulations as a result of increased toxicities and side effects associated with synthetic/orthodox body care products. In the present study, oils obtained via Soxhlet/cold extraction from different underexplored tropical seeds, include: Carica papaya, Dacryodes edulis and Raphia hookeri, were investigated and characterised for their potential sustainable application in skin care formulations. The three oils obtained from the seed samples were also analysed for their fatty acids composition by capillary gas chromatography-mass spectrometry (GC-MS) following transesterification using acid-catalysed hydrolysis. Several in vitro biological activities, include: antibacterial, antifungal, and antityrosinase, were determined using standard procedure. The seed oils from C. papaya, D. edulis, and R. hookeri afforded a yield of 19.89, 8.27 and 0.04%, respectively. The major fatty acids composition of the seed oils from C. papaya were docosanoic (15.36%), elaidic (51.83%), linoleic (17.47%) and stearic (11.22%) acids while D. edulis had palmitic (13.98%), linoleic (50.08%), dihomo-γ-linolenic (15.53%) and oleic acids (10.16%). Palmitic acid (33.88%), elaidic (28.74%), palmitoleic acid (18.98%) and stearic acids (8.57%) were the most prominent in R. hookeri. The antimicrobial activity of the oils investigated at 30 μg/mL revealed that C. papaya significantly inhibited the growth of Saccharomyces cerevisiae, while D. edulis inhibited the growth of Staphylococcus aureus, Rhizopus stolonifera, Penicillium citrinum, Saccharomyces cerevisiae and Aspergillus niger. R. hookeri inhibited the growth of Salmonella typhi, Rhizopus stolonifera, Penicillium citrinum and Saccharomyces cerevisiae. Likewise, C. papaya had an antityrosinase activity with an IC50 value of 0.26 μg/mL, while D. edulis and R. hookeri had an IC50 value of 4.52 and 0.83 μg/mL, respectively. The formulated cream products from the seed oils of C. papaya and D. edulis exhibited dose response activities on the microorganisms and the tyrosinase enzyme. The in silico analysis also re-affirms that the oil components had significant interactions with the tyrosinase enzyme by exhibiting strong affinity via numerous van der Waals forces comparable to the standard, kojic acid. This study has revealed that oils from the seeds of the underutilised plants; C. papaya, D. edulis, and R. hookeri, can be further exploited for medicinal and industrial purposes, particularly in the green cosmetic formulation sector for the regulation of skin pigmentation. However, more studies in animal models would be required to validate the bioactivity and toxicity.
Key Words: Lipid, fatty acid, antimicrobial, in silico, tyrosinase, binding affinity
|
|
|
Introduction
There is an increasing global demand for the adoption of green chemistry for products and product development due to its benign properties. Green Chemistry, also known as Sustainable Chemistry, which involves the creation of chemical products and processes that minimize or eliminate the use of hazardous substances has received global attention in the last few decades. The concept has had a significant impact on businesses, education, the environment, and the general consumer world. The concept is both profitable and beneficial to human health and the environment [1]. Hence, the application of green chemistry in the preparation and manufacture of skin care products forms the fulcrum of recent developments in the cosmetic world [2]. The concept has been promoted recently in the cosmetic sectors for the preparation and production of products that are safe for humans and the environment. Seed oil plays an important role in the production of green cosmetics [2,3]. Among tropical seeds that have been underexplored and underutilised are Carica papaya, Dacryodes edulis, and Raphia hookeri.
Carica papaya of the family Caricacea is a globally renowned plant that produces fleshly edible pulp with high quality vitamins. It contains seeds that are round and dark brown in nature. Like the fruit, the seed is also very rich in nutrients with excellent medicinal properties that can be used to manage a variety of ailments. The leaves, seeds, latex, and fruit of the plant have all been shown to possess significant medicinal value [4]. Despite its medicinal potential, the seed and the seed oil are grossly discarded, under-valued, or ignored. D. edulis of the family Burseraceae is an endemic tropical African plant. The fruit and seed are used as food, fodder, and medicine to cure earache, fever, and headache [5]. D. edulis seeds have been investigated as a source of high-quality oil [6]. R. hookeri (Raphia palm) of the family Arecaceae is a rare tropical tree with characteristic oblong edible pulp but an extremely hard nut when matured and dried [7]. On account of its rare nature, there is a dearth of information on the studies on the plant. Hence, this research aimed to characterize three underexplored tropical seeds (C. papaya, D. edulis, and R. hookeri) and explore their oil for bioactive, eco-friendly, safe cosmetic formulations using the principle of green chemistry.
Chemicals, solvents, and other reagents used were of analytical grade. Where applicable, the solvent was re-distilled before use. L-tyrosine was a product of Sigma-Aldrich, USA, while the microplate spectrophotometer was a Spectra Count, Packard, USA. For centrifugation, a Bench centrifuge Model 800D was used.
Matured C. papaya seeds were obtained within the Ilorin metropolis in Kwara State, while D. edulis seeds were obtained from Owerri in Imo State and R. hookeri from Umuchu, Anambra State, Nigeria. The seeds were identified and authenticated at the herbarium unit of the Plant Biology Department, University of Ilorin, Ilorin, Nigeria. The seeds were dried at ambient temperature, de-shelled, pulverized, and then kept in a cool dark place for further work.
The pulverized seed material was extracted in Soxhlet extractor for 6 hr, as well as cold n-hexane, for three days. The extracts obtained were concentrated in vacuo using the rotary evaporator and the resulting oils were air-dried, stored a in glass vial and kept in a cool dry place for further work. The yield was determined using the expression below:
The physicochemical properties of the oils determine their quality and hence, what the seed oils are suitable for. These properties of the oils, which include acid value, iodine value, saponification value, peroxide value, ester value, density, specific gravity, and pH, were determined using standard procedures with slight modifications where applicable [8 – 11].
Each of the oils (1 g) was weighed into a flask with 25 mL of diethyl ether and 25 mL of methanol. Three drops of phenolphthalein indicator were added. The mixture was warmed in a water bath for 5 minutes and titrated against 0.1 M KOH with constant shaking until the pink colour appeared that indicated the end point [10 – 14]. The acid value of the oil was evaluated using the equation:
where, W = Weight of oil (in grams)
V = Volume of the standard alcoholic potassium hydroxide solution required to neutralize the sample
N = Normality of the solution
Each of the oils (1 g) was weighed into a 250 mL conical flask and the oil was dissolved in 25 mL carbon tetrachloride. Twenty-five mL Wijis solution was added and the mixture allowed to stand in the dark for one hour. The liberated iodine was titrated against 0.1 M sodium thiosulphate using starch indicator [10 – 12, 14] The iodine value was determined using the expression:
where, W = Weight of oil (in grams)
B = Volume of standard sodium thiosulfate solution for blank (in mL)
A = Volume of standard sodium thiosulfate solution required for the sample
N = Normality
where, W0 = Weight (in grams) of empty measuring cylinder
W1 = Weight (in grams) of measuring cylinder with oil
W2 = Weight (in grams) of measuring cylinder with water
A clean and dried measuring cylinder (10 mL) was weighed and recorded as W0. Each oil (1 mL) was measured into the cylinder, weighed and recorded as W1 [12 – 14]. Thereafter, the density was determined by using the formula:
where, W0 = Weight (in grams) of empty measuring cylinder
W1 = Weight (in grams) of measuring cylinder with oil
Each of the oils (0.5 g) was weighed into a flask containing 1 g of potassium iodide and 13 mL glacial acetic acid; 7 mL chloroform was added to it. The conical flask was placed in a water bath for 1 minute, after which 20 mL of 5% potassium iodide mixture and 25 mL of water were added. The mixture was titrated against 0.002 M sodium thiosulphate to attain a colourless solution using a starch indicator. Blank titration was carried out [10 – 14]. The peroxide value was calculated from the expression [15]:
where, W = Weight (in grams) of the oil
N = Normality of Na2S2O3
S = Volume (in mL) of Na2S2O3
Each of the oils (0.5 g) was weighed into a flask containing 25 mL of methanolic KOH and mixed together. The mixture was warmed in a water bath for 5 min and 3 drops of phenolphthalein were added while the contents were titrated against 0.5 M HCl until the pink colour disappeared. The discolouration indicated the end point. A blank titration was performed by omitting the oil (b mL). The saponification value was calculated using the expression [2 – 15]:
where, W = Weight (in grams) of the oil
M = Molarity of HCl
56.1 = Molecular weight of KOH
The Ester value was estimated as the difference between the saponification value and the acid value [10 – 14].
The pH meter was used to determine the level of acidity or basicity of the oil and the formulated cream products.
The oil (2g) was weighed and transferred to a beaker containing 10 mL of 0.2 M methanolic HCl. The mixture was refluxed for 1 hour, poured into a separating funnel and extracted with hexane. The mixture was shaken and allowed to settle down for the two layers to separate. The oil layer was col-lected, concentrated, and air-dried; the oil obtained was kept in glass vials for GC-MS analyses [2].
To determine the fatty acid profile from the seeds of C. papaya, D. edulis and R. hookeri, 1.0 μL of the trans-esterified oil was injected in a non-overlap mode to a Gas Chromatography-Mass Spectrometry GC–MS QP 2010SE Ultra Shimadzu Japan with a FI and selective mass detector 5973 RTx. The GC was equipped with a HP-5MS column with a size of 30 m by 0.25 mm and 0.25 μm film thickness set to pressure flow control mode at 100.0 kPa. The heater and interface were operated at 100 and 300 °C, respectively, while the injection temperature was set at 250 °C. Total flow and column flow were 58.7 and 1.79 mL/min, respectively, as linear velocity was 35.2 cm/sec. Elution was done isothermally using a split ratio of 30:1 at an equilibration time of 3.0 minutes and a purge flow of 3.0 mL/min. The MS parameters included electron impact ionization with electron energy of 70 eV, and mass range of m/z 50–550, using the selective ion monitoring (SIM) mode. The scan was operated for few 25.5 minutes and chemical constituents were identified primarily by comparing the fragmentation pattern of each spectrum with reference compounds in the NIST library.
The UV-Visible analysis of the seed oils was carried out using a VWR UV-6300PC Double Beam Spectrophotometer using n-hexane as the dissolving solvent. The concentration of the stock solution was 30 μg/mL.
The antibacterial and antifungal assays with the minimum inhibitory concentrations were evaluated using standard protocol by determining the zone of inhibition of the oil and cream products [16]. Briefly, for the antibacterial evaluation, the test samples (30 μg/mL each) were prepared and 1 mL each was added to 9 mL of sterile molten Muller Hinton agar (MHA) and potato dextrose agar (PDA), respectively, at 40 °C. The medium was poured into sterile petri dishes and allowed to dry before streaking for 18 hours for selected isolates. The petri dishes were incubated at 37 °C for 24 hours for bacteria growth, while the PDA plates were incubated at ambient temperature, and fungi growth was examined after 72 hours. All the plates were examined for the presence or absence of microbial growth. The minimum inhibition concentration (MIC) was taken as the least concentration that prevents bacterial and fungal growth, respectively.
The tyrosinase inhibition activity potential was carried out following standard protocol [17]. Aliquots (10 μL) of a solution composed of 125 μmL-1 of mushroom tyrosinase (Sigma-Aldrich, USA) were added to 96-well microplates, and then 70 μL of pH 6.8 phosphate buffer solution and 60 μL of the oils (350 μg mL-1 in n-hexane) were also added. For the positive control, 60 μL of kojic acid (17.5 μgmL-1 in n-hexane) was used instead of the seed oil, and for the negative control, 60 μL of n-hexane was used. To the resultant mixture, 70 μL of L-tyrosine (Sigma-Aldrich, USA) was added at a concentration of 0.3 mgmL-1 in distilled water. The absorbance of the microplate wells was read using a microplate spectro-photometer (Spectra Count, Packard, USA) at 510 nm (T0). Then, the microplates were incubated at 30 ± 1°C for 60 min and the absorbance was measured again (T1). An additional incubation period of 60 min at 30 ± 1°C was done and, after this period, a new spectrophotometric reading was taken (T2). The inhibitory percentage at the two time points (T1 and T2) was obtained according to the formula:

|
where IA% = Inhibitory activity
C = Negative control absorbance at 510 nm
S = Sample or positive control absorbance at 510 nm (absorbance at time T1 or T2 minus the absorbance at time T0).
The membrane stabilization activity of the oils and creams was evaluated on bovine red blood cells exposed to both heat and hypotonic induced lyses using standard procedure [18, 19]. Briefly, fresh bovine blood samples were collected into an anticoagulant [containing dextrose (2%), sodium citrate (0.8%), citric acid (0.05%) and sodium chloride (0.42%)]. Blood samples were centrifuged at 3000 rpm on a Bench centrifuge Model 800D for 10 min at room temperature. The supernatants (plasma and leucocytes) were carefully removed while the packed red blood cell was washed in fresh normal saline (0.85% w/v NaCl). The process of washing and centrifugation was repeated five times until the supernatants were clear.
The membrane stabilizing activity assay was carried out using 2% (v/v) bovine erythrocyte suspension while indomethacin was used as the standard drug. The assay mixtures consisted of 2 ml of hyposaline (0.25% w/v) sodium chloride, 1.0 ml of 0.15 M sodium phosphate buffer, pH 7.4, 0.5 ml of 2% (v/v) bovine erythrocyte suspension, 0.0 - 1.0 ml of drugs (standard, extracts/fractions) and final reaction mixtures were made up to 4.5 ml with isosaline. Drugs were omitted in the blood control, while the drug control did not contain the erythrocyte suspension. The reaction mixtures were incubated at 56°C for 30 min on a water bath, followed by centrifugation at 5000 rpm in a Gallenkamp Bench Centrifuge for 10 min at room temperature. While the blood control represents 100% lysis or zero percent stability [18], the absorbance of the released haemoglobin was read at 560 nm. The percentage membrane stability was estimated using the expression:

|
The thin-layer chromatography of the oils was carried out using a pre-coated TLC plate to determine the complexity of components in the extracted oils. The oils were spotted in a TLC plate and developed in an n-hexane and ethyl acetate solvent mixture (3:1 for C. papaya and D. edulis; while 9:1 for R. hookeri). The chromatoplate was viewed under the UV lamp at 254 and 366 nm, respectively.
Molecular docking was adopted as a computational technique used to study the interaction of molecules in the binding sites of target proteins. The goal of ligand-protein docking is to understand the interaction of a ligand with a protein of known three-dimensional structure. Molecular docking calculations are a common assay used to determine the biological activity of molecules in silico. With docking methods, large numbers of molecules are screened at a relatively lower cost than in laboratory experiments. The technique is a key tool in structural molecular biology and computer-assisted drug design [20]. Molecular docking technique was used to investigate the in vitro inhibition effects of C. papaya, D. edulis, and R. hookeri seed oil on tyrosinase enzyme with ID 5M8L. Kojic acid was used as standard. A PDF file was created, the binding site defined and the docking performed following the procedure outlined by Trott and Johnson, 2010 [20].
All materials, which include beeswax and oils from different seeds of C. papaya and D. edulis,were used for the formulation. Beeswax (1 g) was weighed into a 250 mL beaker and melted in a warm water bath. Seed oils (2 g) were added to the beaker and heated for 3 minutes. The mixture was transferred immediately to a container for cooling and solidification. This procedure was repeated while varying the amount of oil and beeswax as indicated (Table 1).
All experiments were performed in replicate except otherwise indicated and the results were presented as mean of the values. For the bioassay, the concentration causing 50% inhibition (IC50) was estimated from a dose-response curve.
Results and Discussion
The percentage yield of oils from the seeds of C. papaya, D. edulis and R. hookeri extracted using n-hexane provided 19.89, 8.27 and 0.04%. The considerable percentage yield of oil from C. papaya showed that it can be exploited for industrial use. D. edulis can also be harnessed while R. hookeri gave a low yield, which might be difficult to be used for any industrial application.
The thin-layer chromatographic results revealed that only oil from the seed of C. papaya shows one distinct component under the UV lamp at 254 nm with Rf value of 0.33. While D. edulis and R. hookeri gave three to five components with different Rf values.
The physicochemical properties of oils from the seeds of C. papaya and D. edulis are as shown (Table 2). Oil from the seeds of R. hookeri was not sufficient for physicochemical analysis because of very low percentage yield.
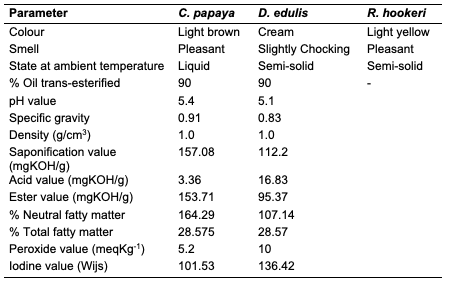 |
The acid value of oils from the seeds of C. papaya and D. edulis were 3.36±0.08 and 16.83±0.00 mg KOH/g, respectively. According to Burla et al., 2018 [22], the acidity of oil suitable for edible purposes should not exceed 4 mg KOH/g. Thus, the oil from the seeds of C. papaya would be suitable for consumption while the oil from the seeds of D. edulis will not. The saponification value of oils from the seeds of C. papaya and D. edulis were relatively low in comparison to those of almond nut (163.39±15.80) and palm kernel seed oil (191.97±3.16 mg KOH/g mg KOH/g). This result indicated that the seed oil contains high molecular weight fatty acids since the saponification values have been reported to be inversely related to the average molecular weight of fatty acids in oil fractions [23]. The saponification value of C. papaya seed oil is 157.08 mg KOH/g, while that of D. edulis seed oil is 112.2 mg KOH/g.
Iodine value is used to measure the degree of unsaturation of the oil. It is useful in studying oxidative rancidity of oils since the higher the unsaturation, the greater the possibility of the oil to go rancid [22]. Oils from the seeds of C. papaya and D. edulis tested had high iodine values (101.53 and 136.42 Wij s, respectively) and are therefore not suitable as non-drying oil. The peroxide value of oils from the seeds of C. papaya and D. edulis obtained were 5.2±0.13 and 10 mEq/kg, respectively. These values were not considered high since crude vegetable oil consists of 10 mEq/kg of peroxide value [22]. The pH value of seed oils from C. papaya and D. edulis (5.4 and 5.1, respectively) were slightly low thereby affirming the acidic nature partly due to acid values. Specific gravity is an important property always considered in oils which serves as feedstock for biodiesel. Denser oils have higher specific gravity. The specific gravity affects the oil properties, particularly the flow and the volatility [22]. The specific gravity of oils from the seeds of C. papaya and D. edulis obtained were 0.91 and 0.83, respectively.
The antimicrobial inhibition potential of the oils and formulations are as depicted (Table 3).
From the data obtained (Table 3), at 30 μg/mL, C. papaya seed oil had little inhibitory effect on the selected bacteria but inhibited Saccharomyces cerevisiae (a fungus) and Candida albicans (a yeast). The formulated creams from C. papaya possess dose response antibacterial activity against Pseudomonas aeruginosa, Streptococcus faecalis, Escherichia coli, Staphylococcus aureus and Salmonella typhi. They also possess dose response antifungal activity on Candida albicans, Rhizopus stolonifera, Penicillium citrinum, Saccharomyces cerevisiae and Aspergillus niger.
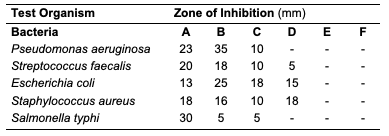 |
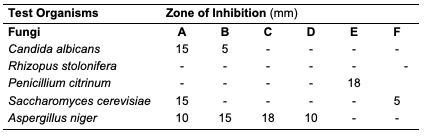 |
Likewise, at 30 μg/mL, D. edulis seed oil (Table 5) inhibited Staphylococcus aureus (a bacterium), Rhizopus stolonifera, Penicillium citrinum, Saccharomyces cerevisiae and Aspergillus niger (fungi). The formulated creams from D. edulis possess dose response antibacterial activity against Pseudomonas aeruginosa, Streptococcus faecalis, Escherichia coli, Staphylococcus aureus and Salmonella typhi. They also possess dose response antifungal activity on Candida albicans, Rhizopus stolonifera, Penicillium citrinum, Saccharomyces cerevisiae and Aspergillus niger.
At 30 μg/mL, R. hookeri inhibited (Table 7) the growth of Salmonella typhi (a bacterium), Rhizopus stolonifera, Penicillium citrinum and Saccharomyces cerevisiae (fungi). The low yield of oil from Raphia hookeri impaired cream formulations.
The trans-esterified seed oils subjected to GC-MS analysis revealed the lipid profile of the oils (Table 10-12). While the major fatty acids contained in C. papaya were docosanoic (15.36%), elaidic (51.83%), linoleic (17.47%) and stearic (11.22%) acids, D. edulis had linoleic (50.08%), palmitic (13.98%), dihomo-γ-linolenic (15.53%) and oleic (10.16%) acids as the major fatty acids. R. hookeri had palmitic (33.88%), elaidic (28.74%), palmitoleic (18.98%) and stearic (8.57%) acids as major component.
The result of the antityrosinase evaluation of the oil samples at different concentrations are as depicted (Table 13).
While all the seed oils exhibited dose-response activities, the antityrosinase assay showed that D. edulis had higher activity than C. papaya and R. hookeri seed oils. R. hookeri seed oils exhibited the lowest activities among all. It is reported that although the antityrosinase activity of the standard, kojic acid was high, it depletes the melanin on the skin and thereby exposes the skin to harmful radiation. From the results, the seed oils have potential to serve as good substitute as applicable in cosmetic production.
From the results above, it is seen that R. hookeri had the lowest IC50 (0.832 μg/mL) when compared to the standard kojic acid (702.55 μg/mL). Hence, R. hookeri oil is not a good tyrosinase inhibitor. The IC50 values of C. papaya and D. edulis had moderate activities (0.2667 and 4.52 μg/mL, respectively).
Human tyrosinase is a copper-containing enzyme in the body that plays a crucial role in the synthesis of the melanin pigment [24, 25]. Tyrosinase is the initiating and rate-limiting enzyme in the synthesis of melanin and is therefore the prime target for anti-melanogenic compounds in cosmetic products. Because of this property of the enzyme, it has physiological roles in the incidence and development of melanoma, a type of skin cancer [26]. Skin disorders such as vitiligo, malignant melanoma, and freckles can all be caused by abnormal tyrosinase expression. Many studies have reported that tyrosinase inhibitors have antioxidant, antibacterial, and antifungal properties, all of which are essential in the treatment of skin diseases [27]. For example, kojic acid, a hyperpigmentation product that binds with the tyrosinase in the skin, inhibits the production of melanin that is needed by the skin and body.
The antityrosinase activity of formulated cream products from C. papaya and D. edulis seed oils was evaluated using a standard protocol. The results obtained are as indicated (Tables 14 and 15). The result indicated that cream products from D. edulis generally had higher antityrosinase activity than that from C. papaya for corresponding formulations, except for product A which had a similar activity trend in both.
It was also noted that both the formulated cream products from C. papaya and D. edulis seed oils exhibited the highest membrane stabilities in comparison to R. hookeri seed oils.
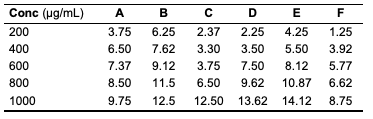 |
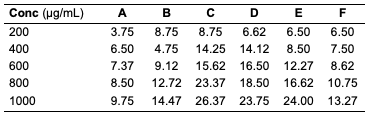 |
In this study, the molecular docking technique was used to investigate the in vitro inhibition effects of C. papaya, D. edulis and R. hookeri seed oil on tyrosinase enzyme with ID 5M8L using kojic acid as standard. Compounds that possess antityrosinase properties are used to reduce hyperpigmentation, reduce spots and induce skin whitening. Conversely, compounds that activate tyrosinase also enhance the synthesis of melanin and ultimately the darkening of the skin. Studies showed that the tyrosinase enzyme contributes to neurodegeneration mechanisms associated with Parkinson's disease and, hence, tyrosinase inhibiting compounds have been studied as a possible therapy for this type of disease.
Some synthetic compounds have been used as antityrosinase drugs to inhibit the enzyme. Kojic acid is one of such compounds. However, the synthetic compounds have adverse effects on both the human skin and the environment. Research has found that kojic acid causes cancer in humans. The search for safe natural alternatives to harmful synthetic compounds has become imperative. Hence, oils from underutilized seeds such as C. papaya and D. edulis may play an important role.
The components identified in C. papaya and D. edulis bound effectively to the tyrosinase on the same target site that kojic acid binds to (Tables 16 and 17). The interactions of the fatty acids with the protein were primarily van der waals (Figure 1).
Using computational techniques, there was structural evidences for the identical binding mode of the oil components and kojic acid in the active site of the human tyrosinase. The molecular docking of the oil components on tyrosinase showed the structural evidence for the identical binding mode of the oil components and kojic acid in the active site of the human tyrosinase. Hence, C. papaya and D. edulis seed oils may be further evaluated as potential alternatives to the implicated kojic acid.
Conclusion
In this study, oils were obtained via Soxhlet and cold extraction from underexplored tropical seeds, which include C. papaya, D. edulis and R. hookeri. The oil yield obtained from the C. papaya, D. edulis and R. hookeri seed were 19.89, 8.27 and 0.04%, respectively. Using an acid-catalysed transesterification reaction, the FAMEs of the seed oils were obtained for lipid profiling. The antimicrobial activity of the oils investigated at 30 μg/mL revealed that C. papaya significantly inhibited the growth of Saccharomyces cerevisiae and Candida albicans, while D. edulis inhibited the growth of Staphylococcus aureus, Rhizopus stolonifera, Penicillium citrinum, Saccharomyces cerevisiae, and Aspergillus niger. R. hookeri inhibited the growth of Salmonella typhi, Rhizopus stolonifera, Penicillium citrinum, and Saccharomyces cerevisiae.
The antityrosinase assay of the oils revealed that seeds of C. papaya had an IC50 value of 0.26 μg/mL, while D. edulis and R. hookeri had an IC50 value of 4.52 and 0.83 μg/mL, respectively. The formulated cream products from the seed oils of C. papaya and D. edulis exhibited dose response activities on the microorganisms and the tyrosinase enzyme. Likewise, the in silico analysis also suggested that the oil components had significant interactions with the tyrosinase enzyme by exhibiting strong affinity via numerous van der waals forces comparable to the standard, kojic acid. The oil may play a remarkable role in the cosmetics or formulations that regulate skin pigmentation.
This study has revealed that oils from the seeds of the underexplored plants; C. papaya, D. edulis and R. hookeri can be further exploited for medicinal and industrial purposes. However, more validation via detailed in vivo studies would be required.
Acknowledgments
The authors acknowledge the support of the Tertiary Education Trust Fund (TETFund) with reference number: UIL/CREDIT/IBRF2014/03 for funding the research. Also, appreciation goes to the CREDIT unit, University of Ilorin for the approval of the research and their cooperation.
Competing Interest
The authors declare no competing interests.
References
- Anastas P, Eghbali N. Green chemistry: Principles and practice. J. R. Soc. Chem., 2010, 39, 301-312. DOI: 10.1039/B918763B.
- Atolani O, Olabiyi ET, Issa AA, Azeez HT, Onoja EG, Ibrahim SO, Zubair MF, Oguntoye OS, Olatunji GA. Green synthesis and characterisation of natural antiseptic soaps from the oils of underutilized tropical seeds. Sustainable Chem. Pharm., 2016, 4, 32-39.
- Zubair MF, Atolani O, Ibrahim SO, Oguntoye OS, Abdulrahim HA, Oyegoke RA, Olatunji GA. Chemical and biological evaluations of potent antiseptic cosmetic products obtained from Momordica charantia seed oil. Sustainable Chem. Pharm., 2018, 9, 35-41.
- Yogiraj V, Goyal PK, Chauhan CS, Goyal A, Vyas B. Carica papaya Linn.: An overview. Int. J. Herb. Med., 2014, 2(5), 01-08.
- Ajayi IA, Adesanwo O. Comparative Study of the mineral element and fatty acid composition of Dacryodes edulis pulp and seed. World J. Agric. Sci., 2009, 5(3), 279-283.
- Arisa NU, Lazarus A. Production and refining of Dacryodes edulis “native pear” seeds oil. Afr. J. Biotechnol., 2008, 7(9), 1684-5315.
- Ogbuagu MN. Vitamins, phytochemicals and toxic elements in the pulp and seed of Raphia palm fruit (Raphia hookeri). Fruits, 2008, 63(5), 297-302. DOI https://doi.org/10.1051/fruits:2008025
- Atolani O, Areh ET, Oguntoye OS, Zubair MF, Fabiyi OA, Oyegoke RA, Tarigha, DE, Adamu N, Adeyemi OS, Kambizi L, Olatunji GA. Chemical composition, antioxidant, anti-lipooxygenase, antimicrobial, anti-parasite and cytotoxicity of Polyalthia longifolia seed oil. Med. Chem. Res., 2019, 28(4), 515-527.
- Atolani O, Adamu N, Oguntoye OS, Zubair MF, Fabiyi OA, Oyegoke RA, Adeyemi OS, Areh ET, Tarigha DE, Kambizi L, Olatunji GA. Chemical characterization, antioxidant, cytotoxicity, Anti-Toxoplasma gondii and antimicrobial potentials of the Citrus sinensis seed oil for sustainable cosmeceutical production. Heliyon, 2020, 6, e03399.
- Gerpen JV. Biodiesel processing and production. Fuel Process. Technol., 2005, 86(10), 1097-1107.
- Ibeto CN, Okoye COB, Ofoefule AU. Comparative study of the physicochemical characterization of some oils as potential feedstock for biodiesel production. Int. Scholarly Res. Network, 2012, 12, 1-5.
- Pearson D in The Chemical Analysis of Food, 7th edition, Church Living-stone, Longman Group Limited, Edinburgh, New York, 1991, 493-494.
- Abdulhamid A, Sani I, Fekal IM. Physicochemical analysis of Soxhlet extracted oils from selected Nigerian seeds. Int. J. Biol. Biomol. Agric. Food Biotechnol. Eng., 2014, 8(11), 1122-1124.
- Kayode ES. Extraction and physicochemical characterization of the oil extract from the seed of umbrella tree (Terminlia mentalis). Int. J. Sci. Eng. Res., 2015, 6(10), 144-147.
- Tsado DB, Ndamitso MM, Ajai AI. Determination of physicochemical properties and fatty acid profile of oil extract of Blighiasapida fruit from selected areas in Niger state, Nigeria. Niger. J. Chem. Res., 2018, 23(1), 21-34.
- Akinpelu DA, Kolawole DO. Phytochemistry and antimicrobial activity of leaf extract of Piliostigma thonningii (Schum). Sci. Focus, 2004, 7, 64-70.
- Macrini DJ, Suffredini IB, Varella AD. Extracts from Amazonian plants have inhibitory activity against tyrosinase: An in vitro evaluation. Braz. J. Pharm. Sci., 2009, 45, 4.
- Oyedapo OO, Sab FC, Olagunju JA. Bioactivity of fresh leaves of Lantana camara. Biomed. Lett., 1999, 59, 175-183.
- Oyedapo OO, Akinpelu BA, Akinwunmi KF, Adeyinka MO, Sipeolu FO. Red blood cell membrane stabilizing potentials of extracts of Lantana camara and its fractions. Int. J. Plant Physiol. Biochem., 2010, 2(4), 46-51.
- Morris GM, Marguerita LW. Molecular modelling of proteins. Springer Link, 2008, 443, 365-382.
- Trott O, Olson AJ. AutoDock Vina: Improving the speed and accuracy of docking with a new scoring function, efficient optimization, and multi-threading. J. Comput. Chem., 2010, 31(2), 455-461.
- Burla S, VS, Malati P, Nancy A, Grace R, Swetha AM, Vivek S. Studies on physicochemical properties & proximate analysis of Carica papaya seed. J. Pharmacogn. Phytochem., 2018, 7(6), 1514-1519.
- Abayeh OJ, Aina EA, Okuonghae CO. Oil content and oil quality characteristics of some Nigerian oil seeds. J. Pure Appl. Sci., 1998, 1, 17-23.
- Ba S, Kumar VV. Recent develop-ments in the use of tyrosinase and laccase in environmental appli-cations. Crit. Rev. Biotechnol., 2017, 37(8), 19-32.
- Kondo T, Hearing VJ. Update on the regulation of mammalian melanocyte function and skin pigmentation. Expert Rev. Dermatol., 2014, 6(1), 97-108.
- Koh HK, Geller AC, Miller DR. Prevention and early detection strategies for melanoma and skin cancer: Current status. Arch. Dermatol., 1996, 132(4), 436-442.
- Sonia S, Kumari LJH, Ruckmani K, Sivakumar M. Antimicrobial and antioxidant potentials of biosynthesized colloidal zinc oxide nanoparticles for a fortified cold cream formulation: A potent nano cosmeceutical application. Mater. Sci. Eng., A, 2017, 79, 581-589.
|